Genomic Mechanisms that Underlie Lack of Recovery of Prince William Sound Herring Following the 1990s Collapse
In the decades following the Exxon Valdez Oil Spill (EVOS), it has become increasingly apparent that oil can be toxic at extremely low concentrations to developing fish embryos including herring, where some toxic phenotypes may be apparent during embryogenesis, but some are delayed until later in life. Therefore, acute and lingering oil may act as an insidious selective force within populations. Much nearshore herring spawning habitat was oiled following the EVOS, and oiling coincided with herring spawning. In subsequent years, lingering oil, which accumulated in nearshore environments and was at least partially bioavailable, may have contributed to impacts in subsequent year classes of developing herring in Prince William Sound (PWS).
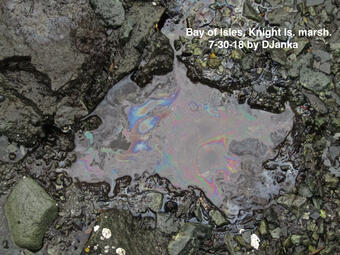
These exposures may have contributed to the herring collapse either through direct delayed toxicity, through the interactive effects of oil and pathogen exposures, or through evolved tolerance to toxicity that came at the cost of compromised immune function. Alternatively, the PWS herring population collapse could have been unrelated to the oil spill, but erosion of genetic diversity associated with the collapse could compromise recovery, compromise resilience to pathogens, or may limit the ability of the population to adapt to future environmental change.
The causes of the herring fishery collapse in PWS in the early 1990’s are complicated and controversial, as is the relationship of the collapse to the 1989 EVOS. We posit that contaminating oil in 1989, and perhaps lingering oil in subsequent years, acted as a selective agent on developing herring, the consequences of which may have contributed to their subsequent decline and/or lack of recovery. Support for this hypothesis emerges from recent discoveries about how exposure to pollutants may drive genetic change in populations of Atlantic killifish (Fundulus heteroclitus): a forage fish with very large population sizes. In particular, some populations of killifish have rapidly evolved within an environment contaminated with creosote, which is chemically and toxicologically similar to crude oil. Creosote pollution acted as a strong selective agent, primarily for survivorship during embryogenesis. Favored were genetic variants with impaired function of the aryl hydrocarbon receptor (AHR) signaling pathway. Normal AHR signaling interacts with immune system signaling, such that impairments in AHR function could come at the cost of normal immune system function. Indeed, in killifish populations we find evidence for subsequent selection on immune system genes, which supports this prediction. These discoveries were achieved through population genomics and comparative transcriptomics and physiological analyses, similar to what we propose here for Pacific herring.
Given these findings in Atlantic killifish, and more generally that pollutants can act as powerful selective agents, that evolutionary genetic change can occur very rapidly, that rapid adaptation often incurs physiological or life history costs, that crude oil can interact with immune function, and that crude oil impairs herring development at extremely low concentrations, we posit the following: 1) Exposure to contaminating oil during 1989 (and perhaps to lingering oil in subsequent years) selected for genetic variants with impaired AHR function in developing embryos. Alternatively (or in addition), exposure to oil in 1989 and perhaps to lingering oil in subsequent years impaired immune function. 2) The frequency of individuals with impaired immune function increased within the PWS population. 3) By 1993, fish from the PWS 1989-year class are recruiting into the fishery, contributing fish with impaired resilience to pathogens, enabling an epizootic, that contributed to the population collapse. 4) Recovery is slow in PWS because compensatory adaptations for immune function is slow, possibly because the collapse significantly eroded genetic diversity available for subsequent natural selection on immune system genes. We design our proposed experiments and contrasts to test this set of hypotheses, as well as to test complementary and alternative hypotheses.
Our discoveries about the nature of pollutant-induced genetic change in Atlantic killifish were enabled through an integrated combination of population genomics, comparative transcriptomics, and comparative physiology studies. Comparative transcriptomics and physiology revealed the functional changes that were induced in pollutant-exposed populations. Population genomics revealed the genetic variants that were under selection, thereby exposing the toxicological mechanisms that were relevant to fish living in polluted sites and exposing the pathways that were also perturbed following the primary adaptation for survival. We propose to deploy a similar toolkit and experimental design to shed light on the causes and consequences of the PWS herring collapse.
This project is the result of a strong collaboration between the Herring Research and Monitoring Program, University of California – Davis, and NOAA – Northwest Fisheries Science Center. Funding partners include the Exxon Valdez Oil Spill Trustee Council and North Pacific Research Board.
In the decades following the Exxon Valdez Oil Spill (EVOS), it has become increasingly apparent that oil can be toxic at extremely low concentrations to developing fish embryos including herring, where some toxic phenotypes may be apparent during embryogenesis, but some are delayed until later in life. Therefore, acute and lingering oil may act as an insidious selective force within populations. Much nearshore herring spawning habitat was oiled following the EVOS, and oiling coincided with herring spawning. In subsequent years, lingering oil, which accumulated in nearshore environments and was at least partially bioavailable, may have contributed to impacts in subsequent year classes of developing herring in Prince William Sound (PWS).
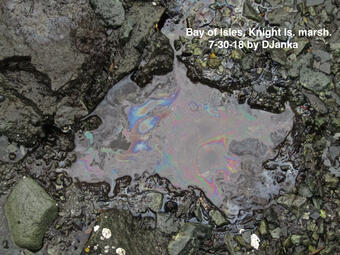
These exposures may have contributed to the herring collapse either through direct delayed toxicity, through the interactive effects of oil and pathogen exposures, or through evolved tolerance to toxicity that came at the cost of compromised immune function. Alternatively, the PWS herring population collapse could have been unrelated to the oil spill, but erosion of genetic diversity associated with the collapse could compromise recovery, compromise resilience to pathogens, or may limit the ability of the population to adapt to future environmental change.
The causes of the herring fishery collapse in PWS in the early 1990’s are complicated and controversial, as is the relationship of the collapse to the 1989 EVOS. We posit that contaminating oil in 1989, and perhaps lingering oil in subsequent years, acted as a selective agent on developing herring, the consequences of which may have contributed to their subsequent decline and/or lack of recovery. Support for this hypothesis emerges from recent discoveries about how exposure to pollutants may drive genetic change in populations of Atlantic killifish (Fundulus heteroclitus): a forage fish with very large population sizes. In particular, some populations of killifish have rapidly evolved within an environment contaminated with creosote, which is chemically and toxicologically similar to crude oil. Creosote pollution acted as a strong selective agent, primarily for survivorship during embryogenesis. Favored were genetic variants with impaired function of the aryl hydrocarbon receptor (AHR) signaling pathway. Normal AHR signaling interacts with immune system signaling, such that impairments in AHR function could come at the cost of normal immune system function. Indeed, in killifish populations we find evidence for subsequent selection on immune system genes, which supports this prediction. These discoveries were achieved through population genomics and comparative transcriptomics and physiological analyses, similar to what we propose here for Pacific herring.
Given these findings in Atlantic killifish, and more generally that pollutants can act as powerful selective agents, that evolutionary genetic change can occur very rapidly, that rapid adaptation often incurs physiological or life history costs, that crude oil can interact with immune function, and that crude oil impairs herring development at extremely low concentrations, we posit the following: 1) Exposure to contaminating oil during 1989 (and perhaps to lingering oil in subsequent years) selected for genetic variants with impaired AHR function in developing embryos. Alternatively (or in addition), exposure to oil in 1989 and perhaps to lingering oil in subsequent years impaired immune function. 2) The frequency of individuals with impaired immune function increased within the PWS population. 3) By 1993, fish from the PWS 1989-year class are recruiting into the fishery, contributing fish with impaired resilience to pathogens, enabling an epizootic, that contributed to the population collapse. 4) Recovery is slow in PWS because compensatory adaptations for immune function is slow, possibly because the collapse significantly eroded genetic diversity available for subsequent natural selection on immune system genes. We design our proposed experiments and contrasts to test this set of hypotheses, as well as to test complementary and alternative hypotheses.
Our discoveries about the nature of pollutant-induced genetic change in Atlantic killifish were enabled through an integrated combination of population genomics, comparative transcriptomics, and comparative physiology studies. Comparative transcriptomics and physiology revealed the functional changes that were induced in pollutant-exposed populations. Population genomics revealed the genetic variants that were under selection, thereby exposing the toxicological mechanisms that were relevant to fish living in polluted sites and exposing the pathways that were also perturbed following the primary adaptation for survival. We propose to deploy a similar toolkit and experimental design to shed light on the causes and consequences of the PWS herring collapse.
This project is the result of a strong collaboration between the Herring Research and Monitoring Program, University of California – Davis, and NOAA – Northwest Fisheries Science Center. Funding partners include the Exxon Valdez Oil Spill Trustee Council and North Pacific Research Board.