Mount Rainier Geology
Mt. Rainier is an active volcano, rising to over 14,000 feet southeast of Seattle.
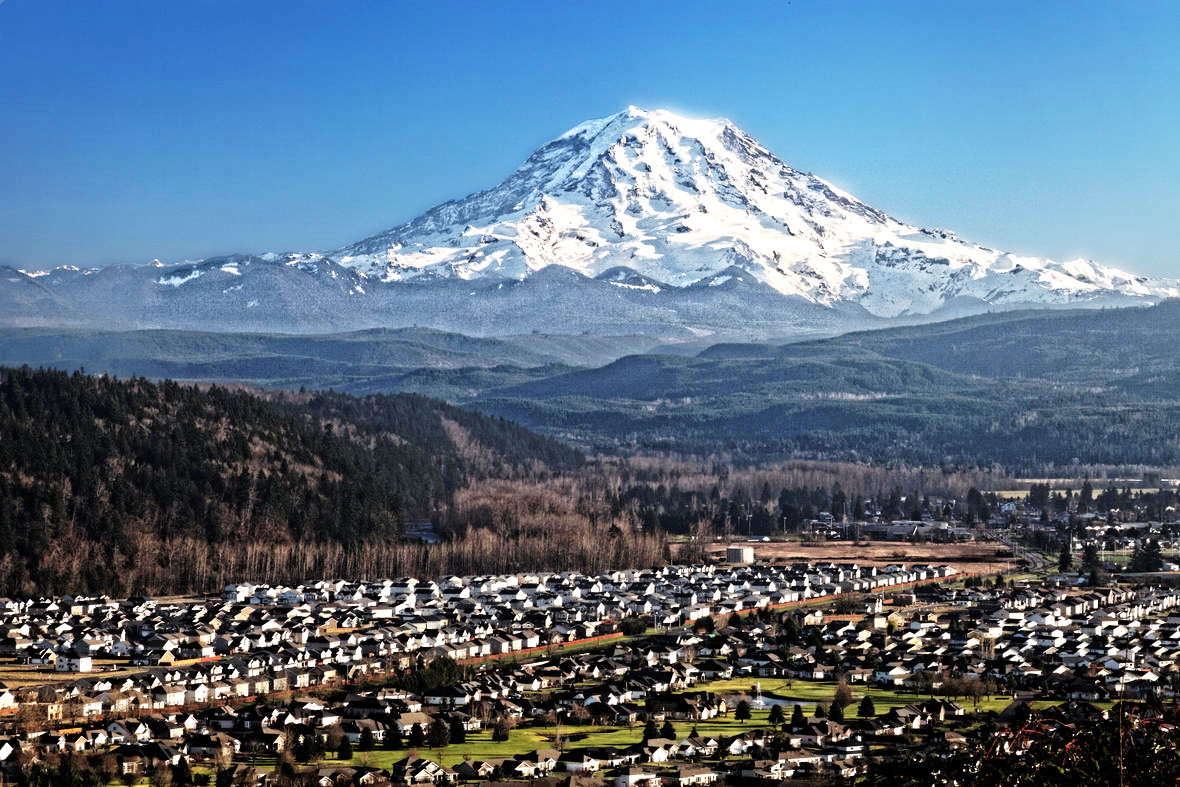
The current edifice, or main portion of the volcano, began its construction of volcano building over the last half million years in the same location the ancestral Mount Rainier stood 1 to 2 million years ago. Before these volcanoes were exposed above ground, large volumes of magma rose, erupted, accumulated, and slowly cooled beneath the surface. Some of this cooled magma, identified as the Tatoosh Granodiorite, can be dated back 18- to 14-million years ago. About 10 million year ago, the western portion of North America was uplifted, and erosional processes washed away the overlying rock exposing the granodiorite below. Rocks observed at the volcano indicate a history of minor pyroclastic flows, or rapidly traveling rock and ash, and hydrothermally altered rocks, or rocks showing alterations due to high temperature water. There are two craters on Mount Rainier that emanate steam and seem to avoid erosion from glaciation, symbolizing a youthful and active volcano with potential of future volcanic eruptions. The volcanism that formed the Mount Rainier we see today ties to its location within the Cascade Range.
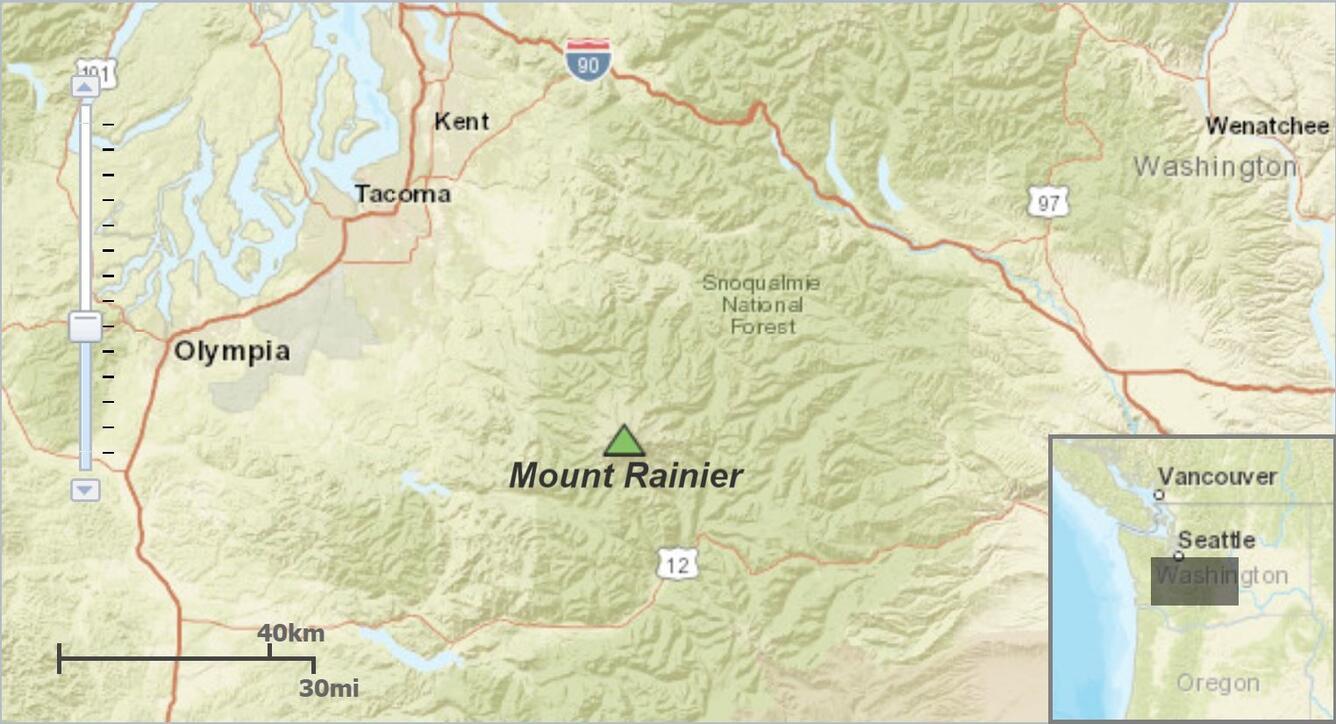
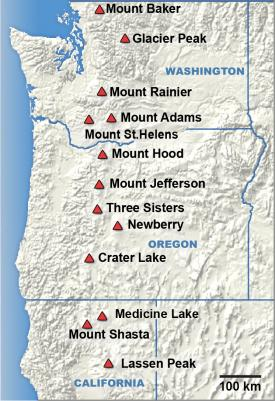
The Cascade Range, in which Mount Rainier is located, is a perfect example of a fundamental concept in geology. It involves a common convergent interaction between tectonic plates where two plates collide and the resulting chain of volcanoes that forms parallel to and inland from the plate boundary. This specific area displays a subduction zone, or a collision zone where a slowly-sliding dense oceanic plate, known as the Juan de Fuca Plate, sinks below the lower density continental North American Plate. As the Juan de Fuca Plate dives deeper into Earth temperatures and pressures increase causing the plate to partially melt and release the less dense material within the rock (water, gases, etc.). The less dense material will then rise, melting and absorbing surrounding rock as it bubbles upwards to form magma. This magma will eventually collect and create magma chambers just below the crust of the earth. These chambers behave similarity to a soda can, staying dormant most of the time unless a sudden disruption occurs. Can you guess what may disturb the balance in the chamber and set off a volcanic eruption? Earthquakes! Just as a sudden and violent shake of a soda can will cause the liquid to explode when opening, volcanoes will react to this quick change in motion and pressure by erupting onto Earth’s surface.
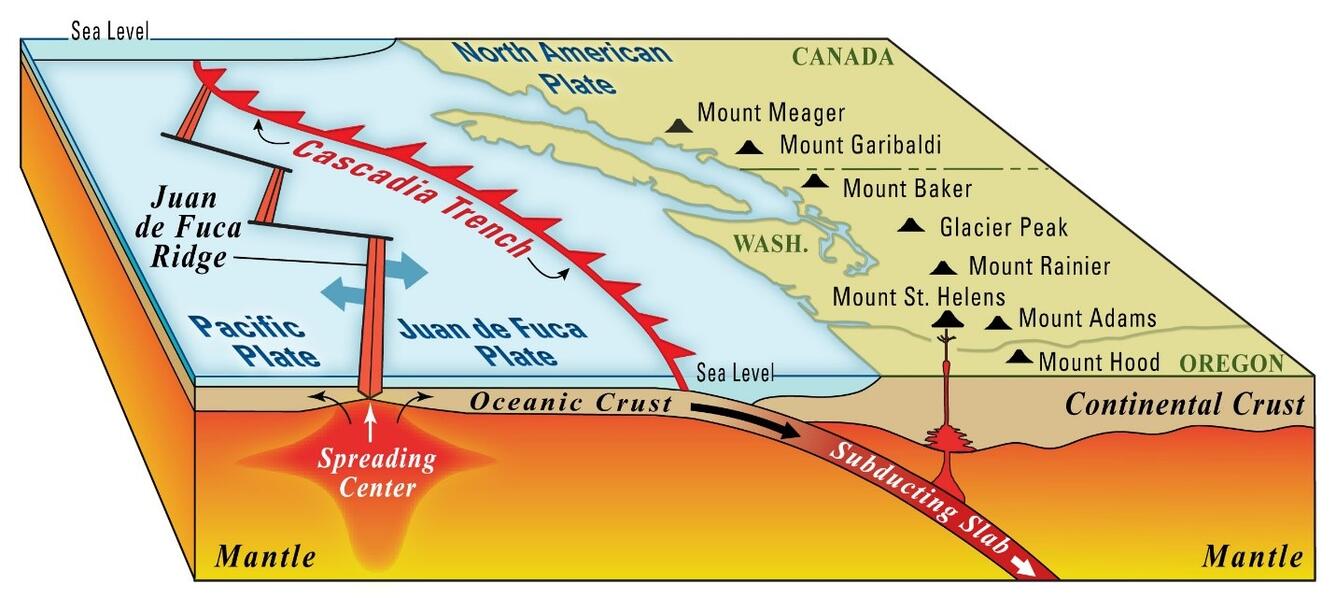
We view volcanic history based on the layers deposited after an explosion. Similar volcanoes such as Crater Lake and Mount St. Helens follow the same set of principles. The stratigraphy, layers of rock strata or beds, acts a time machine for geoscientists to measure, record, and interpret each layer a corresponding time in history. Furthermore, each bed can be measured according to their strike, or overall bearing direction of the bed, and their dip, the angle at which the bed “dips” from horizontal. These measurements allow geologists to get a better sense of the overall orientation of the bed and how it relates to the surrounding formations and landscapes (See more about strike and dip). By examining rocks in the field, scientists can paint a clearer picture of the Earth’s history and anticipate future geologic events. The eruptive history of Mount Rainier is less frequent and less voluminous than surrounding volcanoes due to the magma being more fluid and less sticky during eruption. Its volcanic past dates back 500,000 years ago but has a quieter history when compared to the explosivity of other Cascade volcanoes. Mount Rainier is mainly composed of an accumulation of andesite and dacite lava flows and pumice eruptions. The types of rocks found on this volcano indicate variation in chemical composition when compared to other volcanoes within the region, such as Mount St. Helens.
500 to 420 ka – The first 80,000 years of Mount Rainier’s rock record shows a highly active volcanic period. Thick and well-exposed layers of pyroclastic flows capped by layers of lava flows can be examined dipping radially away from the present-day summit. These steeply dipping beds indicate that the volcano quickly grew to a height similar to what we see today. Ice-chilled columnar remnants of the lava flows can be seen today near the Sunrise visitor facilities.
420 to 280 ka – Rampart: This period left minimal geologic evidence and a reduced rate of lava accumulation due to the infrequent and relatively small eruptions. The Rampart Ridge lava flow is the only record geologists have from the 380,000-year-old eruption, which has since been dramatically eroded by glacial movement.
280 to 160 ka – Mowich: Extensive and rapid lava accumulation took place between 280,000 to 180,000 years ago. Preservation of 200,000-year-old rocks found on the upper west flank indicate that the volcano grew to its maximum height during this period. Increased magma input allowed dikes, or narrow transport pathways cut through weak point of overlying rock, to facilitate large magma movement through volcanic vents onto the surface.
160 to 40 ka – Little Tahoma: Eruptions gradually reduced 160,000 years ago and erosional processes dominated thereafter. Two major lava flow events occurred 130,000 years ago and 105,000 years ago respectively. Both exhibited extremely hot and fluid flow characteristics from sources at great depth, rather than laterally from the Mount Rainier magmatic plumbing system.
40 to 15 ka - Point Success: The rate of eruptions increased during this period leading to a reconstruction of the upper mountain. This period coincides with the last major Ice Age that took place 20,000 years ago, causing a clash of volcanic deposition and glacial erosion.
11 ka to present – Holocene eruption period: The Holocene eruptive history is well preserved in the rock record, identified by the layers of volcanic ash deposited after glacial retreat. Tephra depositional events and lahar flows influenced the topography between 11,000 to 6,700 years ago. A major mudflow event took place about 5,600 years ago, initiated by a collapse of weakened rock containing sufficient water content at the edifice of the volcano. The Osceola Mudflow was 4 km3 in volume, reaching as far west as Puget Sound. This event resulted in a horseshoe-shaped crater open towards the northeast, similar to the crater formed at Mount St. Helens in 1980. The volcano remained relatively quiet until about 2,700 years ago when the onset of eruptions began again. Sequences of tephra falls, pyroclastic flows, lahars and lava flows assisted the growth to the modern-day edifice.
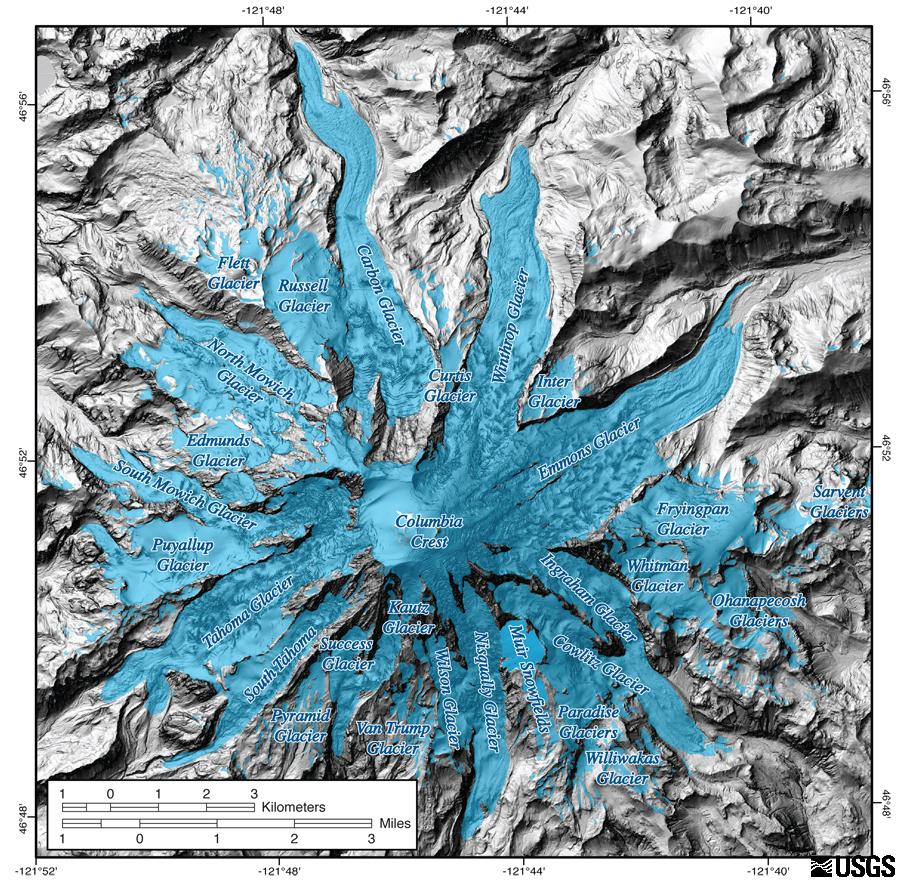
Glaciation has also been an integral part of the creation of the edifice of Mount Rainier. This region was dominated by glaciers both preceding and following the modern cone development. For several periods throughout its volcanic history, glacial erosion fought against the edifice building processes. During times of glacial maximum, the valleys surrounding the edifice were filled with ice extending over 100 km (60 mi) downslope of Mount Rainier. There are two major glacial periods in Mount Rainier’s history:
Hayden Creek -170 to 130 ka: This period involved long valley filling alpine glaciation. Remnants of Hayden Creek till can be found 600 m (nearly 2000 ft) above the adjacent valley floor, indicating that the glaciers were very thick.
Evans Creek -22 to 15 ka: The Evans Creek glaciation was substantially smaller than the glaciers dominating the topography during the Hayden Creek period. The slopes of Mount Rainier were covered by icecaps and all other glaciers further from the volcano were completely confined within valleys.
Two smaller periods of glaciation occurred during the late Pleistocene (12,000 years ago) and at the end of the Little Ice Age (mid-1800’s). The glaciation in the Pacific Northwest was a big influence on the topography of Mount Rainier. The slopes of Mount Rainier were covered with ice and snowpack throughout most of its 500,000-year history. In many cases, the glaciation was so thick that not even the lava could melt through its mass. Instead, the ice cooled the lava from below. This resulted in lava flows being hardened and perched high on the ridges radiating from Mount Rainier’s edifice when the valley-filling glaciers began retreating. Under current conditions, the lava would melt the limited ice cover and eruptions would form destructive lahars. Most of this evidence was gathered by studying the 40,000-year-old Ricksecker Point lava flow, which helped scientists understand the importance of glaciers directing the courses of lava flows at Mount Rainier. Evidence of horizontal movement of lava over glacial terrane is documented in the horizontally oriented columnar joints found at the margin, or boundary, of the Ricksecker Point lava flow.
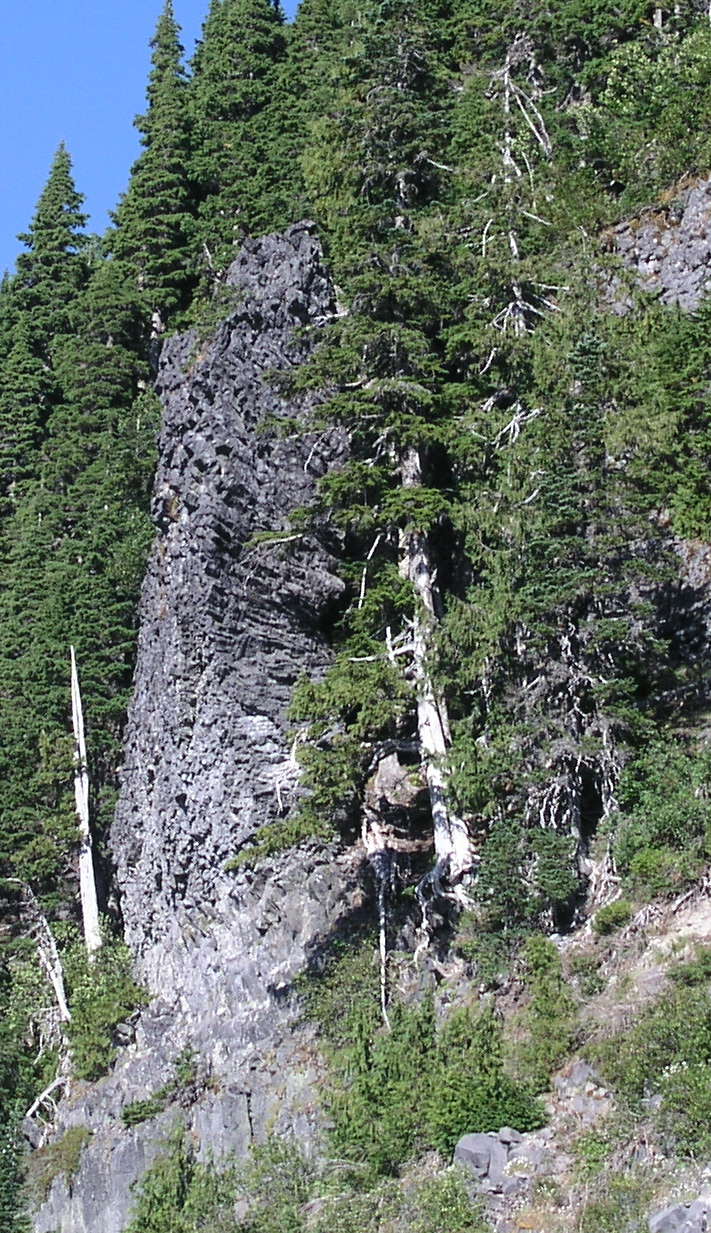
Mount Rainier remains an active volcano, its behavior has been relatively the same over the past half-million years. It will continue to grow, erupt, and collapse as time goes on with volcanic activity being potentially problematic to surrounding residents and air traffic. An eruption would also impact the local scenic and recreational areas and natural resources. When an eruption does occur, there will be warning signs proceeding the major event. Scientists would see changes in their monitoring devices, such as small earthquakes beneath the volcano, subtle land deformation or swelling, and increases in volcanic gas emissions and soil temperatures. Geologists monitor this data to better prepare for future events and how to handle catastrophic events.