Screening toxic compounds using cellular assays
This study is designed to be a high-throughput alternative to traditional methods of using whole fish assays to screen compounds for toxicity. This approach involves an initial pre-screening of a chemical databank to select molecules possessing characteristics identified as predictive criteria for potential toxicity to the fathead minnow, followed by cytotoxicity screening of selected compounds using in vitro cellular assays, and finally in vivo whole fish assays for toxicity screening of chemicals that showed potent cytotoxic effects. This process will allow us to minimize the total number of animals used in experiments, which is ethically preferred.
Although in vivo assays will too be utilized in future projects, only those compounds that show promise as a toxicant will be subjected in order to effectively limit the amount of live fish required for testing. We will use two assays with distinct chemistries that measure different physiological endpoints to assess cytotoxicity. The endpoints are a measure of membrane integrity and cell viability, which are quantified by staining the dead cells’ DNA and a decrease in adenosine triphosphate (ATP), respectively. We will report EC50 values for both assays with the individual compounds, tissue type the cell line used was derived from, and species tested to theoretically identify an invasive carp-selective toxicant.
Introduction
Aquatic resource managers are considerably concerned about controlling invasive fish populations. For example, common carp (Cyprinus carpio), originally brought over from Asia sometime in the 1800s, are now ubiquitous in U.S. waters (Cole 1905). Although they have been around for over 100 years and have become part of U.S. culture, they are still highly detrimental to aquatic ecosystems (Miller and Crowl 2006), so a method to eradicate and control common carp is highly desired (Marking 1992). Also originally from Asia, bigheaded carps [collectively, bighead carp (Hypophthalmichthys nobilis) and silver carp (Hypophthalmichthys molitrix)] were introduced to the U.S. to control plankton in aquaculture ponds and sewage treatment facilities (Rach, Boogaard, and Kolar 2009). In the early 1970s, flooding allowed fish an escape route to natural waterways such as the Mississippi River, where they were able to thrive and spread further along to parts of the Mississippi River drainage basin to become established (Freeze and Henderson 1982). Due to the large size and rapid growth of both bigheaded carp species, which can reach over 50 pounds (Chen et al. 2006), these fish threaten to outcompete native fish species for food, foul the nets of fishermen, and in the case of the silver carp, cause bodily harm by leaping out of the water when disturbed (Vroman 2011). In addition to ecological impacts, a fishing industry of nearly $7 billion, annually, is threatened by bigheaded carps if they were to establish populations in the Great Lakes.
Because of the negative impacts that invasive carp species have on aquatic ecosystems, a method of eliminating or controlling them would be highly valued by aquatic resource managers. Chemical control is an option that is routinely employed to control invasive species. Currently, there are only four registered fish toxicants that are approved for use to control aquatic invasive organisms: the larval lampricides, TFM and niclosamide, and the general fish toxicants, rotenone and antimycin A. The lampricides are used almost exclusively to control sea lamprey populations in the Great Lakes basin, and, in rare cases, have been used for the control of aquatic invertebrates in aquaculture ponds (Applegate and King 1962). Because rotenone and antimycin A are non-selective toxicants, they can be used only in circumstances where a complete eradication of all fishes can be tolerated, such as part of a reclamation project.
Application of a species-specific piscicide is an intuitively appealing approach for controlling invasive fishes, but has rarely been practiced in the U.S. because such selective piscicides are not readily available. Chemical control is known to be expensive, logistically difficult, and unfortunately has been associated with collateral damage to non-target organisms and the environment. Furthermore, although it has high efficacy, it is not 100% effective; retreatment is often required for successful management. Other strategies (e.g., selective harvest, regulatory control, physical barriers, and biological controls) are generally difficult to maintain after the invasion has successfully occurred (Simberloff, Parker, and Windle 2005). Thus, effective management of invasive fishes in the U.S., as well as in other ecosystems, requires the integration of various methods of control into one management program. The use of piscicides, combined with other innovative approaches, such as the use of physical barriers, attractants or repellants, or reproductive inhibitors, applied in an integrated manner to manage invasive fishes may improve the probability of successful renovation of streams and rivers in the U.S.
The selection process of new chemicals to test for species-selective toxicity must be methodical. Quantitative structure-activity relationships (QSARs) are an effective method to predict toxicity to families, classes, or individual species (Dearden 2003) by the use of chemical descriptors. Chemical descriptors, including molecular weight, solubility, and volume (Ballabio et al. 2009), transform chemical information into useful numeric values, which can be used to predict measurable endpoints (Dimitrov et al. 2005). The U.S. EPA has developed a model, named Ecological Structure-Activity Relationship (ECOSAR), which uses the octanol/water partition coefficient (Kow) as the major physical-chemical descriptor for correlating toxic effect (LC50, measured concentration that kills one-half of a group of test animals) of nonreactive neutral organic chemicals, such as dichloromethane and hexane (Könemann 1981, Hermens, Leeuwangh, and Musch 1984). The ECOSARs are linear, mathematical relationships that correlate the log (Kow) and the log LC50 (in mmol/L), and are based on toxicity data for only fathead minnow (Pimephales promelas) at 96-hour static conditions. Scientists at the Upper Midwest Environmental Sciences Center (UMESC) have been focusing their research on expanding the chemical list outside of neutral organic compounds, such as acetic acid and copper (II) sulfate, including models for more than one species of fish and integrating test conditions beyond 96 hours and static type. Previous toxicity reports indicate that bluegill sunfish (Lepomis machrochirus) and rainbow trout (Oncorhynchus mykiss) are more sensitive to most toxic compounds, specifically rotenone and antimycin A (Rach, Boogaard, and Kolar 2009); therefore, toxicity models that incorporate additional fish species is essential. Creating a databank that evaluates species-selective toxicity based on QSARs is of great importance for the development of toxicants specific to invasive aquatic species, such as bighead and silver carp.
The models created at UMESC were used to predict toxicity to three fish species: rainbow trout, bluegill sunfish, and fathead minnow because data to support these models were both available and provided more extensive statistical analysis. Data on the toxicity of chemicals to the bighead carp and silver carp is lacking, so it is not practical to develop models to predict chemical toxicity to these species. Consequently, we chose to use fathead minnow as a surrogate species for the bigheaded carps, as all three species are scientifically classified in the Cyprinidae family.
The selection of chemicals for screening using live fish has significant disadvantages. The most stringent disadvantage is the Interagency Coordinating Committee on the Validation of Alternative Methods (ICCVAM) Authorization Act of 2000, which requires alternative test methods for the determination of chemical toxicity in animals. This act specifies that methods should be revised to reduce the number of animals used and potentially replace animals where applicable. Currently, the Aquatic Ecosystem Health Branch at UMESC has been working with the U.S. Fish & Wildlife Service (FWS) in Onalaska, WI on developing cellular assays to address the ICCVAM act. The cell lines available from the FWS facility can be used as surrogates for live fish in screening a large number of toxic chemicals quickly and determining the most species-selective toxicants.
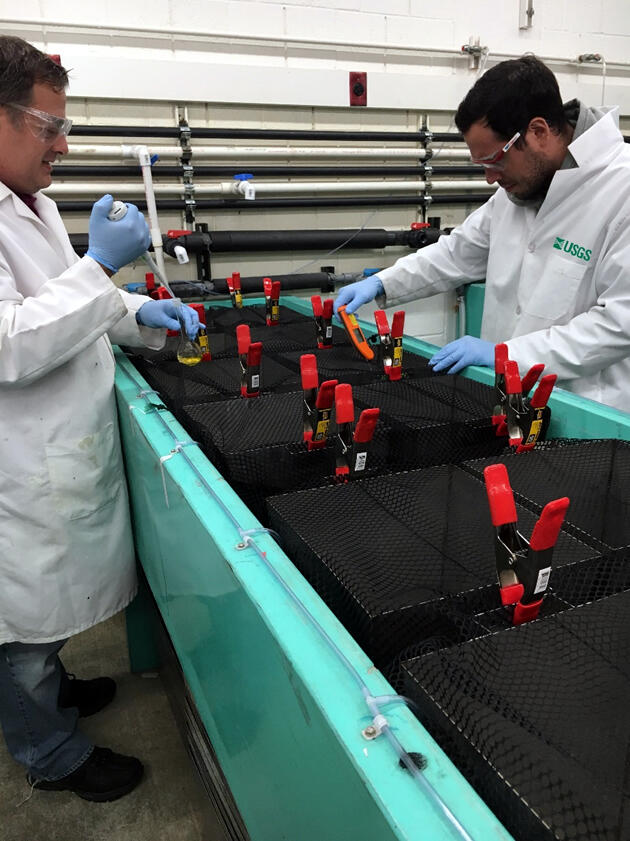
Objective
Test the chemical solutions for species-specific toxicity using cellular assays and cytotoxicity endpoints.
References
American Society for Testing and Materials (ASTM). 2007. Standard Guide for Conducting Acute Toxicity Tests with Fishes, Macroinvertebrates and Amphibians. Annual Book of ASTM Standards. Standard E729-96.
Applegate, Vernon C., and Everett L. King. 1962. “Comparative Toxicity of 3-Trifluormethyl-4-Nitrophenol (TFM) to Larval Lampreys and Eleven Species of Fishes.” Transactions of the American Fisheries Society 91 (4): 342–45.
Ballabio, Davide, Alberto Manganaro, Viviana Consonni, Andrea Mauri, and Roberto Todeschini. 2009. “Introduction to MOLE DB-on-Line Molecular Descriptors Database.” MATCH Commun Math Comput Chem 62: 199–207.
CellTiter-Glo® Technical Bulletin #288, CellTiter-Glo® Luminescent Cell Viability Assay Technical Bulletin #TB288, Promega Corporation, https://www.promega.com/-/media/files/resources/protocols/technical-bulletins/0/celltiter-glo-luminescent-cell-viability-assay-protocol.pdf
CellTox™ Green Technical Manual #375, CellTox™ Green Cytotoxicity Assay Technical Manual #TB375, Promega Corporation, https://www.promega.com/-/media/files/resources/protocols/technical-manuals/101/celltox-green-cytotoxicity-assay-protocol.pdf
Chen, Jun, Ping Xie, Dawen Zhang, Zhixin Ke, and Hua Yang. 2006. “In Situ Studies on the Bioaccumulation of Microcystins in the Phytoplanktivorous Silver Carp (Hypophthalmichthys Molitrix) Stocked in Lake Taihu with Dense Toxic Microcystis Blooms.” Aquaculture 261 (3): 1026–38.
Cole, Leon J. 1905. “The Status of the Carp in America.” Transactions of the American Fisheries Society 34 (1): 201–6.
Dearden, J.C. 2003. In silico prediction of drug toxicity, Journal of Computer-Aided Molecular Design, 17:2-4, 119-127.
Dimitrov, Sabcho, Gergana Dimitrova, Todor Pavlov, Nadezhda Dimitrova, Grace Patlewicz, Jay Niemela, and Ovanes Mekenyan. 2005. “A Stepwise Approach for Defining the Applicability Domain of SAR and QSAR Models.” Journal of Chemical Information and Modeling 45 (4): 839–49.
Freeze, Mike, and Scott Henderson. 1982. “Distribution and Status of the Bighead Carp and Silver Carp in Arkansas.” North American Journal of Fisheries Management 2 (2): 197–200.
Hermens, Joop, Peter Leeuwangh, and Aalt Musch. 1984. “Quantitative Structure-Activity Relationships and Mixture Toxicity Studies of Chloro- and Alkylanilines at an Acute Lethal Toxicity Level to the Guppy (Poecilia Reticulata).” Ecotoxicology and Environmental Safety 8 (4): 388–94.
Könemann, Hans. 1981. “Quantitative Structure-Activity Relationships in Fish Toxicity Studies Part 1: Relationship for 50 Industrial Pollutants.” Toxicology 19 (3): 209–21.
Lennon, Robert E. and Walker, Charles R. 1964. Laboratories and methods for screening fish-control chemcials. U.S. Fish and Wildlife Service.
Marking, Leif L. 1992. “Evaluation of Toxicants for the Control of Carp and Other Nuisance Fishes.” Fisheries 17 (6): 6–13.
Miller, S. A., and T. A. Crowl. 2006. “Effects of Common Carp (Cyprinus Carpio) on Macrophytes and Invertebrate Communities in a Shallow Lake.” Freshwater Biology 51 (1): 85–94.
Rach,J.J., Boogaard, M. and Kolar, C. (2009) Toxicity of Rotenone and Antimycin to Silver Carp and Bighead Carp, North American Journal of Fisheries Management, 29:2, 388-395.
Ritz, C. and Streibig, J.C. (2005) Bioassay Analysis using R. J. Statist. Software, Vol.12:5.
Simberloff, Daniel, Ingrid M. Parker, and Phyllis N. Windle. 2005. “Introduced Species Policy, Management, and Future Research Needs.” Frontiers in Ecology and the Environment 3 (1): 12.
U.S. Food and Drug Administration, Center for Veterinary Medicine, Guidance for Industry, Validation of Analytical Procedures: Methodology, Final Guidance, July 1999.
Vroman, M. E. 2011. “Will Asian Carp Take over the Great Lakes?” In , 375–85.
This study is designed to be a high-throughput alternative to traditional methods of using whole fish assays to screen compounds for toxicity. This approach involves an initial pre-screening of a chemical databank to select molecules possessing characteristics identified as predictive criteria for potential toxicity to the fathead minnow, followed by cytotoxicity screening of selected compounds using in vitro cellular assays, and finally in vivo whole fish assays for toxicity screening of chemicals that showed potent cytotoxic effects. This process will allow us to minimize the total number of animals used in experiments, which is ethically preferred.
Although in vivo assays will too be utilized in future projects, only those compounds that show promise as a toxicant will be subjected in order to effectively limit the amount of live fish required for testing. We will use two assays with distinct chemistries that measure different physiological endpoints to assess cytotoxicity. The endpoints are a measure of membrane integrity and cell viability, which are quantified by staining the dead cells’ DNA and a decrease in adenosine triphosphate (ATP), respectively. We will report EC50 values for both assays with the individual compounds, tissue type the cell line used was derived from, and species tested to theoretically identify an invasive carp-selective toxicant.
Introduction
Aquatic resource managers are considerably concerned about controlling invasive fish populations. For example, common carp (Cyprinus carpio), originally brought over from Asia sometime in the 1800s, are now ubiquitous in U.S. waters (Cole 1905). Although they have been around for over 100 years and have become part of U.S. culture, they are still highly detrimental to aquatic ecosystems (Miller and Crowl 2006), so a method to eradicate and control common carp is highly desired (Marking 1992). Also originally from Asia, bigheaded carps [collectively, bighead carp (Hypophthalmichthys nobilis) and silver carp (Hypophthalmichthys molitrix)] were introduced to the U.S. to control plankton in aquaculture ponds and sewage treatment facilities (Rach, Boogaard, and Kolar 2009). In the early 1970s, flooding allowed fish an escape route to natural waterways such as the Mississippi River, where they were able to thrive and spread further along to parts of the Mississippi River drainage basin to become established (Freeze and Henderson 1982). Due to the large size and rapid growth of both bigheaded carp species, which can reach over 50 pounds (Chen et al. 2006), these fish threaten to outcompete native fish species for food, foul the nets of fishermen, and in the case of the silver carp, cause bodily harm by leaping out of the water when disturbed (Vroman 2011). In addition to ecological impacts, a fishing industry of nearly $7 billion, annually, is threatened by bigheaded carps if they were to establish populations in the Great Lakes.
Because of the negative impacts that invasive carp species have on aquatic ecosystems, a method of eliminating or controlling them would be highly valued by aquatic resource managers. Chemical control is an option that is routinely employed to control invasive species. Currently, there are only four registered fish toxicants that are approved for use to control aquatic invasive organisms: the larval lampricides, TFM and niclosamide, and the general fish toxicants, rotenone and antimycin A. The lampricides are used almost exclusively to control sea lamprey populations in the Great Lakes basin, and, in rare cases, have been used for the control of aquatic invertebrates in aquaculture ponds (Applegate and King 1962). Because rotenone and antimycin A are non-selective toxicants, they can be used only in circumstances where a complete eradication of all fishes can be tolerated, such as part of a reclamation project.
Application of a species-specific piscicide is an intuitively appealing approach for controlling invasive fishes, but has rarely been practiced in the U.S. because such selective piscicides are not readily available. Chemical control is known to be expensive, logistically difficult, and unfortunately has been associated with collateral damage to non-target organisms and the environment. Furthermore, although it has high efficacy, it is not 100% effective; retreatment is often required for successful management. Other strategies (e.g., selective harvest, regulatory control, physical barriers, and biological controls) are generally difficult to maintain after the invasion has successfully occurred (Simberloff, Parker, and Windle 2005). Thus, effective management of invasive fishes in the U.S., as well as in other ecosystems, requires the integration of various methods of control into one management program. The use of piscicides, combined with other innovative approaches, such as the use of physical barriers, attractants or repellants, or reproductive inhibitors, applied in an integrated manner to manage invasive fishes may improve the probability of successful renovation of streams and rivers in the U.S.
The selection process of new chemicals to test for species-selective toxicity must be methodical. Quantitative structure-activity relationships (QSARs) are an effective method to predict toxicity to families, classes, or individual species (Dearden 2003) by the use of chemical descriptors. Chemical descriptors, including molecular weight, solubility, and volume (Ballabio et al. 2009), transform chemical information into useful numeric values, which can be used to predict measurable endpoints (Dimitrov et al. 2005). The U.S. EPA has developed a model, named Ecological Structure-Activity Relationship (ECOSAR), which uses the octanol/water partition coefficient (Kow) as the major physical-chemical descriptor for correlating toxic effect (LC50, measured concentration that kills one-half of a group of test animals) of nonreactive neutral organic chemicals, such as dichloromethane and hexane (Könemann 1981, Hermens, Leeuwangh, and Musch 1984). The ECOSARs are linear, mathematical relationships that correlate the log (Kow) and the log LC50 (in mmol/L), and are based on toxicity data for only fathead minnow (Pimephales promelas) at 96-hour static conditions. Scientists at the Upper Midwest Environmental Sciences Center (UMESC) have been focusing their research on expanding the chemical list outside of neutral organic compounds, such as acetic acid and copper (II) sulfate, including models for more than one species of fish and integrating test conditions beyond 96 hours and static type. Previous toxicity reports indicate that bluegill sunfish (Lepomis machrochirus) and rainbow trout (Oncorhynchus mykiss) are more sensitive to most toxic compounds, specifically rotenone and antimycin A (Rach, Boogaard, and Kolar 2009); therefore, toxicity models that incorporate additional fish species is essential. Creating a databank that evaluates species-selective toxicity based on QSARs is of great importance for the development of toxicants specific to invasive aquatic species, such as bighead and silver carp.
The models created at UMESC were used to predict toxicity to three fish species: rainbow trout, bluegill sunfish, and fathead minnow because data to support these models were both available and provided more extensive statistical analysis. Data on the toxicity of chemicals to the bighead carp and silver carp is lacking, so it is not practical to develop models to predict chemical toxicity to these species. Consequently, we chose to use fathead minnow as a surrogate species for the bigheaded carps, as all three species are scientifically classified in the Cyprinidae family.
The selection of chemicals for screening using live fish has significant disadvantages. The most stringent disadvantage is the Interagency Coordinating Committee on the Validation of Alternative Methods (ICCVAM) Authorization Act of 2000, which requires alternative test methods for the determination of chemical toxicity in animals. This act specifies that methods should be revised to reduce the number of animals used and potentially replace animals where applicable. Currently, the Aquatic Ecosystem Health Branch at UMESC has been working with the U.S. Fish & Wildlife Service (FWS) in Onalaska, WI on developing cellular assays to address the ICCVAM act. The cell lines available from the FWS facility can be used as surrogates for live fish in screening a large number of toxic chemicals quickly and determining the most species-selective toxicants.
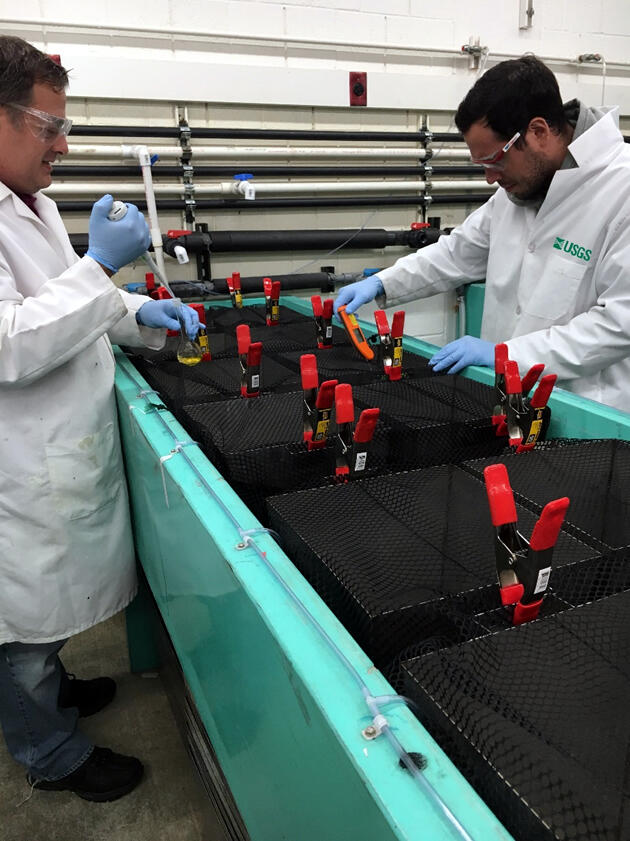
Objective
Test the chemical solutions for species-specific toxicity using cellular assays and cytotoxicity endpoints.
References
American Society for Testing and Materials (ASTM). 2007. Standard Guide for Conducting Acute Toxicity Tests with Fishes, Macroinvertebrates and Amphibians. Annual Book of ASTM Standards. Standard E729-96.
Applegate, Vernon C., and Everett L. King. 1962. “Comparative Toxicity of 3-Trifluormethyl-4-Nitrophenol (TFM) to Larval Lampreys and Eleven Species of Fishes.” Transactions of the American Fisheries Society 91 (4): 342–45.
Ballabio, Davide, Alberto Manganaro, Viviana Consonni, Andrea Mauri, and Roberto Todeschini. 2009. “Introduction to MOLE DB-on-Line Molecular Descriptors Database.” MATCH Commun Math Comput Chem 62: 199–207.
CellTiter-Glo® Technical Bulletin #288, CellTiter-Glo® Luminescent Cell Viability Assay Technical Bulletin #TB288, Promega Corporation, https://www.promega.com/-/media/files/resources/protocols/technical-bulletins/0/celltiter-glo-luminescent-cell-viability-assay-protocol.pdf
CellTox™ Green Technical Manual #375, CellTox™ Green Cytotoxicity Assay Technical Manual #TB375, Promega Corporation, https://www.promega.com/-/media/files/resources/protocols/technical-manuals/101/celltox-green-cytotoxicity-assay-protocol.pdf
Chen, Jun, Ping Xie, Dawen Zhang, Zhixin Ke, and Hua Yang. 2006. “In Situ Studies on the Bioaccumulation of Microcystins in the Phytoplanktivorous Silver Carp (Hypophthalmichthys Molitrix) Stocked in Lake Taihu with Dense Toxic Microcystis Blooms.” Aquaculture 261 (3): 1026–38.
Cole, Leon J. 1905. “The Status of the Carp in America.” Transactions of the American Fisheries Society 34 (1): 201–6.
Dearden, J.C. 2003. In silico prediction of drug toxicity, Journal of Computer-Aided Molecular Design, 17:2-4, 119-127.
Dimitrov, Sabcho, Gergana Dimitrova, Todor Pavlov, Nadezhda Dimitrova, Grace Patlewicz, Jay Niemela, and Ovanes Mekenyan. 2005. “A Stepwise Approach for Defining the Applicability Domain of SAR and QSAR Models.” Journal of Chemical Information and Modeling 45 (4): 839–49.
Freeze, Mike, and Scott Henderson. 1982. “Distribution and Status of the Bighead Carp and Silver Carp in Arkansas.” North American Journal of Fisheries Management 2 (2): 197–200.
Hermens, Joop, Peter Leeuwangh, and Aalt Musch. 1984. “Quantitative Structure-Activity Relationships and Mixture Toxicity Studies of Chloro- and Alkylanilines at an Acute Lethal Toxicity Level to the Guppy (Poecilia Reticulata).” Ecotoxicology and Environmental Safety 8 (4): 388–94.
Könemann, Hans. 1981. “Quantitative Structure-Activity Relationships in Fish Toxicity Studies Part 1: Relationship for 50 Industrial Pollutants.” Toxicology 19 (3): 209–21.
Lennon, Robert E. and Walker, Charles R. 1964. Laboratories and methods for screening fish-control chemcials. U.S. Fish and Wildlife Service.
Marking, Leif L. 1992. “Evaluation of Toxicants for the Control of Carp and Other Nuisance Fishes.” Fisheries 17 (6): 6–13.
Miller, S. A., and T. A. Crowl. 2006. “Effects of Common Carp (Cyprinus Carpio) on Macrophytes and Invertebrate Communities in a Shallow Lake.” Freshwater Biology 51 (1): 85–94.
Rach,J.J., Boogaard, M. and Kolar, C. (2009) Toxicity of Rotenone and Antimycin to Silver Carp and Bighead Carp, North American Journal of Fisheries Management, 29:2, 388-395.
Ritz, C. and Streibig, J.C. (2005) Bioassay Analysis using R. J. Statist. Software, Vol.12:5.
Simberloff, Daniel, Ingrid M. Parker, and Phyllis N. Windle. 2005. “Introduced Species Policy, Management, and Future Research Needs.” Frontiers in Ecology and the Environment 3 (1): 12.
U.S. Food and Drug Administration, Center for Veterinary Medicine, Guidance for Industry, Validation of Analytical Procedures: Methodology, Final Guidance, July 1999.
Vroman, M. E. 2011. “Will Asian Carp Take over the Great Lakes?” In , 375–85.