Temperature-dependent toxicity of molluscicides to zebra mussels
Zebra mussels (Dreissenia polymorpha) are native to the Black, Caspian, and Aral Seas of eastern Europe (Gollasch and Leppäkoski 1999) and they were likely introduced into Lake Erie as veliger larvae in the summer or fall of 1985 (Hebert et al. 1989). Their high reproductive capacity and planktonic larval stage enable zebra mussels to rapidly disperse (Birnbaum 2011). Less than 10 years after introduction, zebra mussels were established in all of the Great Lakes and in the Arkansas, Cumberland, Hudson, Illinois, Mississippi, Ohio, and Tennessee rivers. Additionally, zebra mussels have been reported within the borders or in adjacent waterways of twenty states (Benson et al. 2016). Many pathways have been implicated as overland dispersal mechanisms for transporting zebra mussels to inland lakes, including small, trailed watercraft (Gollash and Leppäkoski 1999). Once established in a waterbody, the likelihood of spreading to nearby waterbodies is greatly enhanced by the “dispersion kernel” phenomenon which is a function of the infestation probability and distance from a source population (Havel et al. 2015).
As of April 2016, the Minnesota Department of Natural Resources had 243 waterbodies listed as infested due to either confirmed zebra mussel presence or interconnection to a waterbody with a confirmed presence (http://www.dnr.state.mn.us/invasives/ais/infested.html, accessed July 8, 2016).
The myriad of ecosystem level impacts that zebra mussels can inflict on naïve systems include dramatic increases in benthic algae and macrophyte biomass as well as increases of >2000% in total zoobenthic biomass (Higgins and Vander Zanden 2010). Zebra mussel attachment and psuedofeces deposition have resulted in interstitial space occlusion and subsequent habitat rejection by spawning lake trout, rendering once productive spawning shoals severely degraded (Einhouse et al. 2013, Marsden and Chotkowski 2001). Due to their sedentary life style and their evolution in dreissenid-free waters, native Unionid mussels are particularly vulnerable to zebra mussel infestations. Zebra mussels have caused severe declines in Unionid mussel abundance with infestation levels as low as 10 zebra mussels/Unionid. The result has been extirpation of Unionid mussels in some waterways following zebra mussel infestation (Napela 1994, Ricciardi et al. 1996, Ricciardi et al. 1998). Of equal concern is the influence that zebra mussels may have on harmful algal blooms (HABS) through selective rejection of Microcystis and other graze-resistant cyanobacteria species (Vanderploeg et al. 2001). HABS pose potential human health hazards as well as causing domestic and wild animal mortalities (Wynne et al. 2015).
Additionally, the economic burden associated with damages and biofouling control costs from zebra mussel infestations in water intake pipes, water filtration systems, and electrical generating facilities has been staggering. Pimentel et al. (2005) cites costs of $1 billion/year in North America, related to damage from and control of biofouling zebra mussels and their close relative, the quagga mussel (D. rostriformis bugensis).
Upon introduction to a new environment, invasive species typically exhibit a population growth lag phase prior to a period of rapid growth (Crooks and Soulé 1999). After this initial lag phase and establishment of the invasive species, eradication is nearly impossible (Bax 2001, Crooks and Soulé 1999). Therefore, early detection and rapid response (EDRR) to an invasive species introduction are paramount for successful eradication (Anderson 2005, Locke and Hanson 2009, Westbrooks, 2004). High fecundity, a planktonic larval stage, and rapid reproductive maturity are factors that increase the probability of unintentional transport and rapid establishment of zebra mussels into uninfested waters. The combination of high dispersal probability and negative impacts that zebra mussels impart on naïve aquatic ecosystems has resulted in zebra mussels being placed on the list of the World’s top 100 worst invasive species (Lowe et al. 2000).
The few successful zebra mussel eradications that have been completed have included: (1) a treatment of a 12-acre quarry lake (Millbrook Quarry, VA) with a potassium chloride solution, (2) hand removal through the use of SCUBA divers in a low calcium lake (Lake George, NY), and (3) dewatering/freeze out of a 255-acre lake (Zorinsky Lake, NE). Two recent EDRR attempts to eradicate zebra mussels in Minnesota were conducted in 2014 and 2015 in Christmas Lake and in Lake Independence. However, eradication attempts were hindered by the lack of knowledge concerning water-temperature dependent treatments. The lack of knowledge impacted the decisions surrounding choice of molluscicide and dosing regimens. Costa et al. (2008) compared the LC50 values of reference toxicants to zebra mussels over the course of a year and found that water temperature was the major factor in determining sensitivity to toxicants. Since the probability of detecting new infestations is likely higher in late summer to autumn when settled veligers have had time to increase in size, the, lack of knowledge of temperature dependence and of concentration/exposure time relationships for molluscicides in general are major impediments to zebra mussel eradication.
Development of temperature-dependent treatment data will lead to the development of a critically needed decision support tool for (1) selecting the correct molluscicide for a proposed rapid-response action, (2) determining the treatment parameters required at specific water temperatures, (3) determining the probability of success for a proposed rapid response action, and (4) estimating treatment related costs of proposed rapid response actions.
Currently, two products (Zequanox® and Earthtec QZ®) are registered by the United States Environmental Protection Agency (USEPA) for zebra mussel control and two additional chemicals have known molluscicidal activity (potassium and niclosamide). The efficacy of Zequanox, a killed-cell bacteria-based control agent, for potential use in open-water zebra mussel control applications has been investigated (Luoma et al., 2015). However, temperature was not included as a test variable in that investigation.
Earthtec QZ, a copper-based product, has been demonstrated to be effective for killing both zebra and quagga mussels. While temperature ranges for this product were not evaluated (Claudi et al. 2014, Watters et al. 2013) temperature is known to influence copper ion toxicity in zebra mussels (Rao and Khan 2000). Potassium was discovered to be toxic to zebra mussels in the early 1990’s during an attempt to standardize molluscicide toxicity tests by using USEPA standard reconstituted reference water (Fisher et al. 1991). Further evaluation of potassium, as potassium chloride, found that it exhibited the most selectivity for zebra mussels out of 18 potential molluscicides (Waller et al. 1993). Muriate of Potash (MOP), which contains approximately 99% potassium chloride, was used to eradicate zebra mussels from a highly infested 12-acre, 93-feet deep quarry lake in Virginia. Approximately 174,000 gallons of a MOP solution were applied to Millbrook Quarry over the course of 3 weeks to achieve and maintain a target concentration of 100 mg/L (http://www.dgif.virginia.gov/zebramussels, accessed 1/19/2016). Limited literature was found that specifically evaluated the effects of water temperature on toxicity of potassium to zebra mussels. Costa et al. (2008) evaluated the effects of water temperature on the toxicity of potassium to zebra mussels, however, only 48-h LC50 values were reported. The demonstrated toxicity of potassium to zebra mussels and the documented successful use of potassium (as MOP) to eradicate an established population of zebra mussels warrant further investigation into the effects of temperature and concentration on the toxicity of potassium to zebra mussels.
Bayluscide® products contain the ethanolamine salt of niclosamide (2-Aminoethanol salt of 2',5-dichloro-4'-nitrosalicylanilide) as the active ingredient. Various formulations of Bayluscide have been registered with the USEPA for sea lamprey control including a 3.2% granule, a 20% emulsifiable concentrate, and a 70% wettable powder (WP). Bayluscide 70% WP is also USEPA-registered to control snails in aquaculture ponds. Waller et al. (1993) found Bayluscide 70% WP to be highly toxic to and somewhat selective for zebra mussels. The selectivity ratio (lowest nontarget LC50/highest zebra mussel LC50) was 2.18 when compared to the toxicity to two species of freshwater fish and a freshwater mussel. The USEPA registration status of products containing niclosamide as an active ingredient, combined with the demonstrated toxicity of niclosamide to zebra mussels, warrants further investigation of its use as a rapid response tool for localized zebra mussel infestations.
A comprehensive evaluation is needed on the efficacy of both registered molluscicides and top candidate molluscicidal compounds at various water temperatures. Water temperature specific data on molluscicide dose and duration of exposure required to achieve complete zebra mussel mortality is essential for managers that need to quickly select the appropriate molluscicide and implement a rapid response to kill zebra mussels in localized infestations or potentially in small water bodies.
Objective
- Develop water-temperature specific rapid response treatment protocols for the complete kill of localized zebra mussel infestations which include: (1) molluscicide selection, (2) treatment concentration, and (3) treatment duration. Specifically, for each of four products/chemicals (Zequanox®, Earthtec QZ®, potassium chloride, and niclosamide), we will evaluate the dose and duration of exposure required to achieve a complete kill of zebra mussels at four water temperatures ranging from ~7 to 22°C.
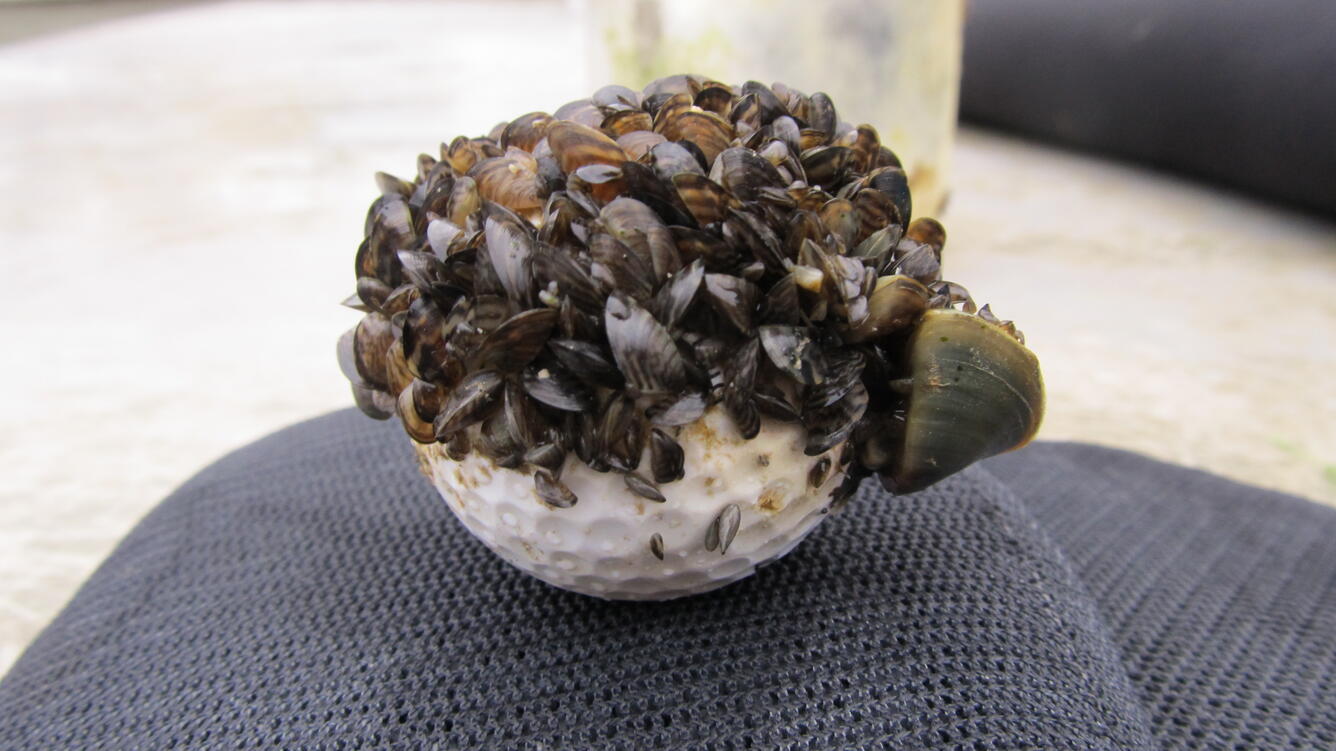
References
Anderson, L.W., 2005, California’s Reaction to Caulerpa taxifolia: A Model for Invasive Species Rapid Response. Biological Invasions, 7(6), pp.1003-1016.
ASTM International, 2013, ASTM Standard E2455-06 (2013)—Standard guide for conducting laboratory toxicity tests with freshwater mussels: West Conshohocken, Pa., ASTM International, 52 p.
Bax, N., Carlton, J.T., Mathews‐Amos, A., Haedrich, R.L., Howarth, F.G., Purcell, J.E., Rieser, A. and Gray, A., 2001. The control of biological invasions in the world's oceans. Conservation Biology, 15(5), pp.1234-1246.
Benson, A.J., Raikow, D., Larson, J., Fusaro, A., and Bogdanoff, A.K., 2016, Dreissena polymorpha. USGS Nonindigenous Aquatic Species Database, Gainesville, FL.
http://nas.er.usgs.gov/queries/FactSheet.aspx?speciesID=5 Revision Date: 6/26/2014.
Birnbaum, Christina, 2011, NOBANIS—Invasive alien species fact sheet—Dreissena polymorpha: Online Database of the European Network on Invasive Alien Species, accessed January 22, 2016 at http://www.nobanis.org/files/factsheets/Dreissena_polymorpha.pdf.
Claudi, R., Prescott, T.H., Mastisky, S. and Coffey, H., 2014, Efficacy of copper based algaecides for control of Quagga and Zebra Mussels. Report for California department of water resources, aquatic nuisance species program, pp.1-58.
Costa, R., Aldridge, D.C. and Moggridge, G.D., 2008, Seasonal variation of zebra mussel susceptibility to molluscicidal agents. Journal of applied ecology, 45(6), pp.1712-1721.
Crooks, J.A. and Soulé, M.E., 1999, Lag times in population explosions of invasive species: causes and implications. Invasive species and biodiversity management, pp.103-125.
Einhouse, D.W., Markham, J.L.,Todd, M.T., Wilkinson, M.A., Zeller, D.L., Zimar, R.C., and Beckwith B.J., 2013, NYS DEC Lake Erie 2012 Annual Report. New York State Department of Environment Conservation, Albany, New York, USA.
Fisher, S.W., Stromberg, P., Bruner, K.A. and Boulet, L.D., 1991, Molluscicidal activity of potassium to the zebra mussel, Dreissena polymorphia: toxicity and mode of action. Aquatic Toxicology, 20(4), pp.219-234.
Gollasch, S. and Leppäkoski, E. (eds), 1999, Initial risk assessment of alien species in Nordic coastal waters. Nordic Council of Ministers, Copenhagen in Birnbaum, C. (2011): NOBANIS – Invasive Alien Species Fact Sheet – Dreissena polymorpha. – From: Online Database of the European Network on Invasive Alien Species – NOBANIS www.nobanis.org, Date of access 1/21/2016.
Havel, J.E., Kovalenko, K.E., Thomaz, S.M., Amalfitano, S. and Kats, L.B., 2015, Aquatic invasive species: challenges for the future. Hydrobiologia, 750(1), pp.147-170.
Hebert, P. D., Muncaster, B., and G. Mackie, G., 1989, Ecological and genetic studies on Dreissena polymorpha (Pallas): a new mollusc in the Great Lakes. Canadian Journal of Fisheries and Aquatic Sciences 46:1587-1591.
Higgins, S.N. and Zanden, M.V., 2010, What a difference a species makes: a meta-analysis of dreissenid mussel impacts on freshwater ecosystems. Ecological monographs, 80(2), pp.179-196.
Kolar, C.S. and Lodge, D.M., 2000, Freshwater nonindigenous species: interactions with other global changes. Invasive species in a changing world, pp.3-30.
Locke, A. and Hanson, J.M., 2009, Rapid response to non-indigenous species. 1. Goals and history of rapid response in the marine environment. In Aquatic Invasions (Vol. 4, No. 1, pp. 237-247). European Research Network on Invasive Species.
Lowe, S., Browne, M., Boudjelas, S. and De Poorter, M., 2000, 100 of the world's worst invasive alien species: a selection from the global invasive species database (p. 12). Auckland,, New Zealand: Invasive Species Specialist Group.
Luoma, J.A., Severson, T.J., Weber, K.L., and Mayer, D.A., 2015, Efficacy of Pseudomonas fluorescens (Pf-CL145A) spray dried powder for controlling zebra mussels adhering to test substrates: U.S. Geological Survey Open-File Report 2015–1050, 519 p., http://dx.doi.org/10.3133/ofr20151050.
Mackie, G.L. and Claudi, R., 2009, Monitoring and control of macrofouling mollusks in fresh water systems. CRC Press.
Marsden, J E.., and Chotkowski, M.A., 2001, Lake trout spawning on artificial reefs and the effect of zebra mussels: fatal attraction? Journal of Great Lakes Research, 27(1), 33-43.
Mooney, H.A. and Hobbs, R.J., 2000, Global change and invasive species: where do we go from here. Invasive Species in a Changing World. Washington, DC: Island, pp.425-434.
Nalepa, T.F., 1994, Decline of native unionid bivalves in Lake St. Clair after infestation by the zebra mussel, Dreissena polymorpha. Canadian Journal of Fisheries and Aquatic Sciences, 51(10), pp.2227-2233.
Pimentel, D., Zuniga, R. and Morrison, D., 2005, Update on the environmental and economic costs associated with alien-invasive species in the United States. Ecological economics, 52(3), pp.273-288.
Rao, D. G. V. P., and Khan, M.A.Q., 2000, Zebra mussels: enhancement of copper toxicity by high temperature and its relationship with respiration and metabolism. Water Environment Research 72:175-178.
Ricciardi, A., Whoriskey, F.G. and Rasmussen, J.B., 1996, Impact of the (Dreissena) invasion on native unionid bivalves in the upper St. Lawrence River. Canadian Journal of Fisheries and Aquatic Sciences, 53(6), pp.1434-1444.
Ricciardi, A., Neves, R.J. and Rasmussen, J.B., 1998, Impending extinctions of North American freshwater mussels (Unionoida) following the zebra mussel (Dreissena polymorpha) invasion. Journal of Animal Ecology, pp.613-619.
SAS, 2010, Version 9.3: Cary, N.C., SAS Institute Inc.
Vanderploeg, H.A., Liebig, J.R., Carmichael, W.W., Agy, M.A., Johengen, T.H., Fahnenstiel, G.L. and Nalepa, T.F., 2001, Zebra mussel (Dreissena polymorpha) selective filtration promoted toxic Microcystis blooms in Saginaw Bay (Lake Huron) and Lake Erie. Canadian Journal of Fisheries and Aquatic Sciences, 58(6), pp.1208-1221.
Waller, DL, Rach, JJ, Cope, WG, Marking, LL, Fisher, SW & Dabrowska, H 1993, Toxicity of candidate molluscicides to zebra mussels (Dreissena polymorpha) and selected nontarget organisms. Journal of Great Lakes Research. 4 edn, vol. 19: 695-702.
Watters, A., Gerstenberger, S.L. and Wong, W.H., 2013, Effectiveness of EarthTec® for killing invasive quagga mussels (Dreissena rostriformis bugensis) and preventing their colonization in the Western United States. Biofouling, 29(1), pp.21-28.
Westbrooks, R.G., 2004, New approaches for early detection and rapid response to invasive plants in the United States. Weed Technology, 18(sp1), pp.1468-1471.
Wynne, T.T., Davis, T., Kelty, R., Anderson, E. and Joshi, S.J., NOAA Forecasts and Monitors Blooms of Toxic Cyanobacteria in Lake Erie. http://www.glerl.noaa.gov/pubs/fulltext/2015/20150041.pdf, accessed 1/21/2016.
Zebra mussels (Dreissenia polymorpha) are native to the Black, Caspian, and Aral Seas of eastern Europe (Gollasch and Leppäkoski 1999) and they were likely introduced into Lake Erie as veliger larvae in the summer or fall of 1985 (Hebert et al. 1989). Their high reproductive capacity and planktonic larval stage enable zebra mussels to rapidly disperse (Birnbaum 2011). Less than 10 years after introduction, zebra mussels were established in all of the Great Lakes and in the Arkansas, Cumberland, Hudson, Illinois, Mississippi, Ohio, and Tennessee rivers. Additionally, zebra mussels have been reported within the borders or in adjacent waterways of twenty states (Benson et al. 2016). Many pathways have been implicated as overland dispersal mechanisms for transporting zebra mussels to inland lakes, including small, trailed watercraft (Gollash and Leppäkoski 1999). Once established in a waterbody, the likelihood of spreading to nearby waterbodies is greatly enhanced by the “dispersion kernel” phenomenon which is a function of the infestation probability and distance from a source population (Havel et al. 2015).
As of April 2016, the Minnesota Department of Natural Resources had 243 waterbodies listed as infested due to either confirmed zebra mussel presence or interconnection to a waterbody with a confirmed presence (http://www.dnr.state.mn.us/invasives/ais/infested.html, accessed July 8, 2016).
The myriad of ecosystem level impacts that zebra mussels can inflict on naïve systems include dramatic increases in benthic algae and macrophyte biomass as well as increases of >2000% in total zoobenthic biomass (Higgins and Vander Zanden 2010). Zebra mussel attachment and psuedofeces deposition have resulted in interstitial space occlusion and subsequent habitat rejection by spawning lake trout, rendering once productive spawning shoals severely degraded (Einhouse et al. 2013, Marsden and Chotkowski 2001). Due to their sedentary life style and their evolution in dreissenid-free waters, native Unionid mussels are particularly vulnerable to zebra mussel infestations. Zebra mussels have caused severe declines in Unionid mussel abundance with infestation levels as low as 10 zebra mussels/Unionid. The result has been extirpation of Unionid mussels in some waterways following zebra mussel infestation (Napela 1994, Ricciardi et al. 1996, Ricciardi et al. 1998). Of equal concern is the influence that zebra mussels may have on harmful algal blooms (HABS) through selective rejection of Microcystis and other graze-resistant cyanobacteria species (Vanderploeg et al. 2001). HABS pose potential human health hazards as well as causing domestic and wild animal mortalities (Wynne et al. 2015).
Additionally, the economic burden associated with damages and biofouling control costs from zebra mussel infestations in water intake pipes, water filtration systems, and electrical generating facilities has been staggering. Pimentel et al. (2005) cites costs of $1 billion/year in North America, related to damage from and control of biofouling zebra mussels and their close relative, the quagga mussel (D. rostriformis bugensis).
Upon introduction to a new environment, invasive species typically exhibit a population growth lag phase prior to a period of rapid growth (Crooks and Soulé 1999). After this initial lag phase and establishment of the invasive species, eradication is nearly impossible (Bax 2001, Crooks and Soulé 1999). Therefore, early detection and rapid response (EDRR) to an invasive species introduction are paramount for successful eradication (Anderson 2005, Locke and Hanson 2009, Westbrooks, 2004). High fecundity, a planktonic larval stage, and rapid reproductive maturity are factors that increase the probability of unintentional transport and rapid establishment of zebra mussels into uninfested waters. The combination of high dispersal probability and negative impacts that zebra mussels impart on naïve aquatic ecosystems has resulted in zebra mussels being placed on the list of the World’s top 100 worst invasive species (Lowe et al. 2000).
The few successful zebra mussel eradications that have been completed have included: (1) a treatment of a 12-acre quarry lake (Millbrook Quarry, VA) with a potassium chloride solution, (2) hand removal through the use of SCUBA divers in a low calcium lake (Lake George, NY), and (3) dewatering/freeze out of a 255-acre lake (Zorinsky Lake, NE). Two recent EDRR attempts to eradicate zebra mussels in Minnesota were conducted in 2014 and 2015 in Christmas Lake and in Lake Independence. However, eradication attempts were hindered by the lack of knowledge concerning water-temperature dependent treatments. The lack of knowledge impacted the decisions surrounding choice of molluscicide and dosing regimens. Costa et al. (2008) compared the LC50 values of reference toxicants to zebra mussels over the course of a year and found that water temperature was the major factor in determining sensitivity to toxicants. Since the probability of detecting new infestations is likely higher in late summer to autumn when settled veligers have had time to increase in size, the, lack of knowledge of temperature dependence and of concentration/exposure time relationships for molluscicides in general are major impediments to zebra mussel eradication.
Development of temperature-dependent treatment data will lead to the development of a critically needed decision support tool for (1) selecting the correct molluscicide for a proposed rapid-response action, (2) determining the treatment parameters required at specific water temperatures, (3) determining the probability of success for a proposed rapid response action, and (4) estimating treatment related costs of proposed rapid response actions.
Currently, two products (Zequanox® and Earthtec QZ®) are registered by the United States Environmental Protection Agency (USEPA) for zebra mussel control and two additional chemicals have known molluscicidal activity (potassium and niclosamide). The efficacy of Zequanox, a killed-cell bacteria-based control agent, for potential use in open-water zebra mussel control applications has been investigated (Luoma et al., 2015). However, temperature was not included as a test variable in that investigation.
Earthtec QZ, a copper-based product, has been demonstrated to be effective for killing both zebra and quagga mussels. While temperature ranges for this product were not evaluated (Claudi et al. 2014, Watters et al. 2013) temperature is known to influence copper ion toxicity in zebra mussels (Rao and Khan 2000). Potassium was discovered to be toxic to zebra mussels in the early 1990’s during an attempt to standardize molluscicide toxicity tests by using USEPA standard reconstituted reference water (Fisher et al. 1991). Further evaluation of potassium, as potassium chloride, found that it exhibited the most selectivity for zebra mussels out of 18 potential molluscicides (Waller et al. 1993). Muriate of Potash (MOP), which contains approximately 99% potassium chloride, was used to eradicate zebra mussels from a highly infested 12-acre, 93-feet deep quarry lake in Virginia. Approximately 174,000 gallons of a MOP solution were applied to Millbrook Quarry over the course of 3 weeks to achieve and maintain a target concentration of 100 mg/L (http://www.dgif.virginia.gov/zebramussels, accessed 1/19/2016). Limited literature was found that specifically evaluated the effects of water temperature on toxicity of potassium to zebra mussels. Costa et al. (2008) evaluated the effects of water temperature on the toxicity of potassium to zebra mussels, however, only 48-h LC50 values were reported. The demonstrated toxicity of potassium to zebra mussels and the documented successful use of potassium (as MOP) to eradicate an established population of zebra mussels warrant further investigation into the effects of temperature and concentration on the toxicity of potassium to zebra mussels.
Bayluscide® products contain the ethanolamine salt of niclosamide (2-Aminoethanol salt of 2',5-dichloro-4'-nitrosalicylanilide) as the active ingredient. Various formulations of Bayluscide have been registered with the USEPA for sea lamprey control including a 3.2% granule, a 20% emulsifiable concentrate, and a 70% wettable powder (WP). Bayluscide 70% WP is also USEPA-registered to control snails in aquaculture ponds. Waller et al. (1993) found Bayluscide 70% WP to be highly toxic to and somewhat selective for zebra mussels. The selectivity ratio (lowest nontarget LC50/highest zebra mussel LC50) was 2.18 when compared to the toxicity to two species of freshwater fish and a freshwater mussel. The USEPA registration status of products containing niclosamide as an active ingredient, combined with the demonstrated toxicity of niclosamide to zebra mussels, warrants further investigation of its use as a rapid response tool for localized zebra mussel infestations.
A comprehensive evaluation is needed on the efficacy of both registered molluscicides and top candidate molluscicidal compounds at various water temperatures. Water temperature specific data on molluscicide dose and duration of exposure required to achieve complete zebra mussel mortality is essential for managers that need to quickly select the appropriate molluscicide and implement a rapid response to kill zebra mussels in localized infestations or potentially in small water bodies.
Objective
- Develop water-temperature specific rapid response treatment protocols for the complete kill of localized zebra mussel infestations which include: (1) molluscicide selection, (2) treatment concentration, and (3) treatment duration. Specifically, for each of four products/chemicals (Zequanox®, Earthtec QZ®, potassium chloride, and niclosamide), we will evaluate the dose and duration of exposure required to achieve a complete kill of zebra mussels at four water temperatures ranging from ~7 to 22°C.
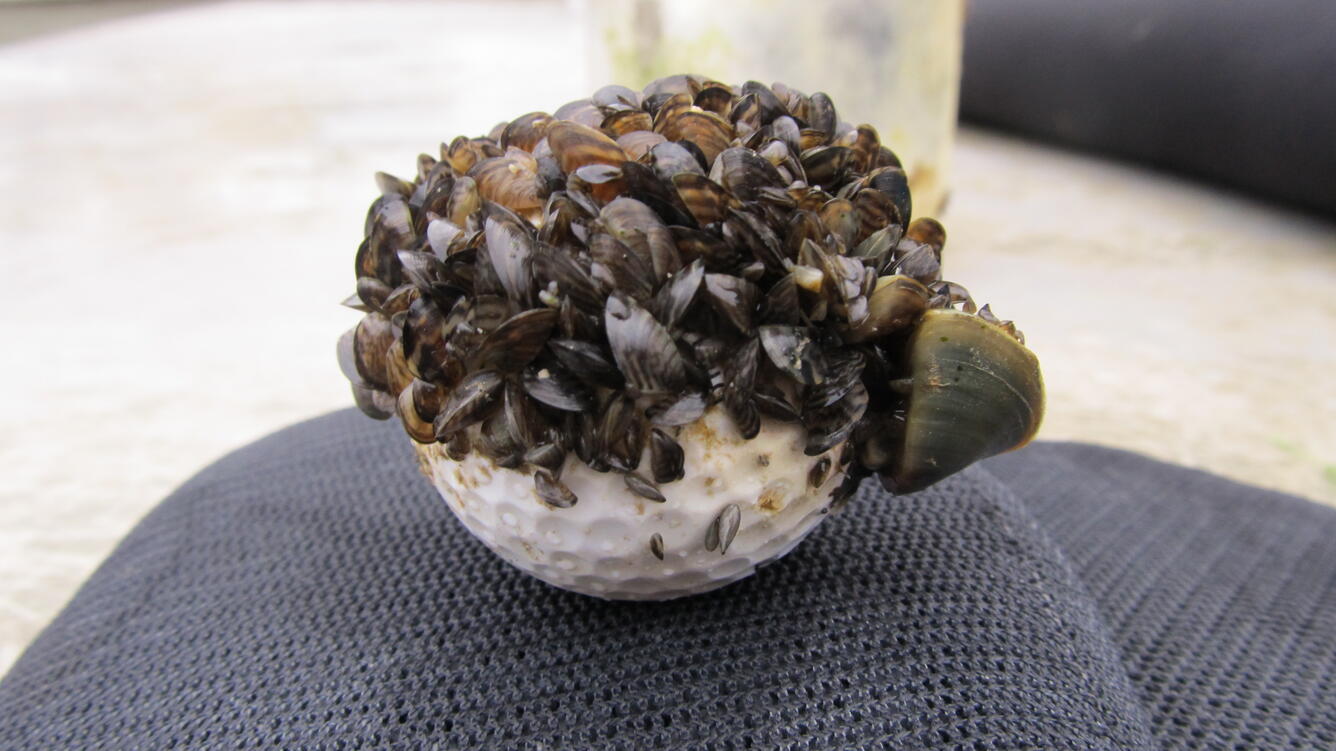
References
Anderson, L.W., 2005, California’s Reaction to Caulerpa taxifolia: A Model for Invasive Species Rapid Response. Biological Invasions, 7(6), pp.1003-1016.
ASTM International, 2013, ASTM Standard E2455-06 (2013)—Standard guide for conducting laboratory toxicity tests with freshwater mussels: West Conshohocken, Pa., ASTM International, 52 p.
Bax, N., Carlton, J.T., Mathews‐Amos, A., Haedrich, R.L., Howarth, F.G., Purcell, J.E., Rieser, A. and Gray, A., 2001. The control of biological invasions in the world's oceans. Conservation Biology, 15(5), pp.1234-1246.
Benson, A.J., Raikow, D., Larson, J., Fusaro, A., and Bogdanoff, A.K., 2016, Dreissena polymorpha. USGS Nonindigenous Aquatic Species Database, Gainesville, FL.
http://nas.er.usgs.gov/queries/FactSheet.aspx?speciesID=5 Revision Date: 6/26/2014.
Birnbaum, Christina, 2011, NOBANIS—Invasive alien species fact sheet—Dreissena polymorpha: Online Database of the European Network on Invasive Alien Species, accessed January 22, 2016 at http://www.nobanis.org/files/factsheets/Dreissena_polymorpha.pdf.
Claudi, R., Prescott, T.H., Mastisky, S. and Coffey, H., 2014, Efficacy of copper based algaecides for control of Quagga and Zebra Mussels. Report for California department of water resources, aquatic nuisance species program, pp.1-58.
Costa, R., Aldridge, D.C. and Moggridge, G.D., 2008, Seasonal variation of zebra mussel susceptibility to molluscicidal agents. Journal of applied ecology, 45(6), pp.1712-1721.
Crooks, J.A. and Soulé, M.E., 1999, Lag times in population explosions of invasive species: causes and implications. Invasive species and biodiversity management, pp.103-125.
Einhouse, D.W., Markham, J.L.,Todd, M.T., Wilkinson, M.A., Zeller, D.L., Zimar, R.C., and Beckwith B.J., 2013, NYS DEC Lake Erie 2012 Annual Report. New York State Department of Environment Conservation, Albany, New York, USA.
Fisher, S.W., Stromberg, P., Bruner, K.A. and Boulet, L.D., 1991, Molluscicidal activity of potassium to the zebra mussel, Dreissena polymorphia: toxicity and mode of action. Aquatic Toxicology, 20(4), pp.219-234.
Gollasch, S. and Leppäkoski, E. (eds), 1999, Initial risk assessment of alien species in Nordic coastal waters. Nordic Council of Ministers, Copenhagen in Birnbaum, C. (2011): NOBANIS – Invasive Alien Species Fact Sheet – Dreissena polymorpha. – From: Online Database of the European Network on Invasive Alien Species – NOBANIS www.nobanis.org, Date of access 1/21/2016.
Havel, J.E., Kovalenko, K.E., Thomaz, S.M., Amalfitano, S. and Kats, L.B., 2015, Aquatic invasive species: challenges for the future. Hydrobiologia, 750(1), pp.147-170.
Hebert, P. D., Muncaster, B., and G. Mackie, G., 1989, Ecological and genetic studies on Dreissena polymorpha (Pallas): a new mollusc in the Great Lakes. Canadian Journal of Fisheries and Aquatic Sciences 46:1587-1591.
Higgins, S.N. and Zanden, M.V., 2010, What a difference a species makes: a meta-analysis of dreissenid mussel impacts on freshwater ecosystems. Ecological monographs, 80(2), pp.179-196.
Kolar, C.S. and Lodge, D.M., 2000, Freshwater nonindigenous species: interactions with other global changes. Invasive species in a changing world, pp.3-30.
Locke, A. and Hanson, J.M., 2009, Rapid response to non-indigenous species. 1. Goals and history of rapid response in the marine environment. In Aquatic Invasions (Vol. 4, No. 1, pp. 237-247). European Research Network on Invasive Species.
Lowe, S., Browne, M., Boudjelas, S. and De Poorter, M., 2000, 100 of the world's worst invasive alien species: a selection from the global invasive species database (p. 12). Auckland,, New Zealand: Invasive Species Specialist Group.
Luoma, J.A., Severson, T.J., Weber, K.L., and Mayer, D.A., 2015, Efficacy of Pseudomonas fluorescens (Pf-CL145A) spray dried powder for controlling zebra mussels adhering to test substrates: U.S. Geological Survey Open-File Report 2015–1050, 519 p., http://dx.doi.org/10.3133/ofr20151050.
Mackie, G.L. and Claudi, R., 2009, Monitoring and control of macrofouling mollusks in fresh water systems. CRC Press.
Marsden, J E.., and Chotkowski, M.A., 2001, Lake trout spawning on artificial reefs and the effect of zebra mussels: fatal attraction? Journal of Great Lakes Research, 27(1), 33-43.
Mooney, H.A. and Hobbs, R.J., 2000, Global change and invasive species: where do we go from here. Invasive Species in a Changing World. Washington, DC: Island, pp.425-434.
Nalepa, T.F., 1994, Decline of native unionid bivalves in Lake St. Clair after infestation by the zebra mussel, Dreissena polymorpha. Canadian Journal of Fisheries and Aquatic Sciences, 51(10), pp.2227-2233.
Pimentel, D., Zuniga, R. and Morrison, D., 2005, Update on the environmental and economic costs associated with alien-invasive species in the United States. Ecological economics, 52(3), pp.273-288.
Rao, D. G. V. P., and Khan, M.A.Q., 2000, Zebra mussels: enhancement of copper toxicity by high temperature and its relationship with respiration and metabolism. Water Environment Research 72:175-178.
Ricciardi, A., Whoriskey, F.G. and Rasmussen, J.B., 1996, Impact of the (Dreissena) invasion on native unionid bivalves in the upper St. Lawrence River. Canadian Journal of Fisheries and Aquatic Sciences, 53(6), pp.1434-1444.
Ricciardi, A., Neves, R.J. and Rasmussen, J.B., 1998, Impending extinctions of North American freshwater mussels (Unionoida) following the zebra mussel (Dreissena polymorpha) invasion. Journal of Animal Ecology, pp.613-619.
SAS, 2010, Version 9.3: Cary, N.C., SAS Institute Inc.
Vanderploeg, H.A., Liebig, J.R., Carmichael, W.W., Agy, M.A., Johengen, T.H., Fahnenstiel, G.L. and Nalepa, T.F., 2001, Zebra mussel (Dreissena polymorpha) selective filtration promoted toxic Microcystis blooms in Saginaw Bay (Lake Huron) and Lake Erie. Canadian Journal of Fisheries and Aquatic Sciences, 58(6), pp.1208-1221.
Waller, DL, Rach, JJ, Cope, WG, Marking, LL, Fisher, SW & Dabrowska, H 1993, Toxicity of candidate molluscicides to zebra mussels (Dreissena polymorpha) and selected nontarget organisms. Journal of Great Lakes Research. 4 edn, vol. 19: 695-702.
Watters, A., Gerstenberger, S.L. and Wong, W.H., 2013, Effectiveness of EarthTec® for killing invasive quagga mussels (Dreissena rostriformis bugensis) and preventing their colonization in the Western United States. Biofouling, 29(1), pp.21-28.
Westbrooks, R.G., 2004, New approaches for early detection and rapid response to invasive plants in the United States. Weed Technology, 18(sp1), pp.1468-1471.
Wynne, T.T., Davis, T., Kelty, R., Anderson, E. and Joshi, S.J., NOAA Forecasts and Monitors Blooms of Toxic Cyanobacteria in Lake Erie. http://www.glerl.noaa.gov/pubs/fulltext/2015/20150041.pdf, accessed 1/21/2016.