Influence of Sea-Level Rise on Wetland Vegetation Community Structure, Primary Productivity, Organic Matter Decomposition and Carbon Storage Completed
This study will employ a space for time substitution to show long-term effects of rising sea-level and increasing salinity on vegetation community structure, primary production and decomposition. Productivity and decomposition rates will be estimated for four wetland plant community types defined by salinity zones and dominant plant species.
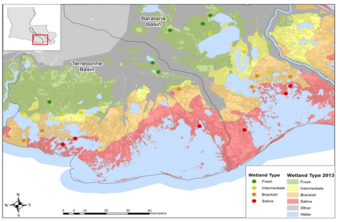
Science Issue and Relevance: Coastal areas comprise less than 20% of the total land area in the continental United States, yet they are home to over 50% of the nation’s population. High population densities in these regions often lead to severe environmental degradation as a result of flood control measures, commercial navigation support, and fossil fuels extraction. Effective restoration and management of these valuable coastal areas is of paramount importance, particularly in an age of changing climate.
Relative sea level rise rates have been observed in some regions of the northern Gulf of Mexico in excess of 10 mm/yr. Pulsed fluvial inputs which once nourished wetlands of the Mississippi River delta plain have been virtually eliminated for nearly a century because of the hydrologic separation of containment levees. Impoundment effects resulting from the construction of oil and gas pipeline canals and spoil banks have altered flooding regimes, and deep channel access has increased tidal energy and salinity through enhanced estuary-ocean exchange. Although the relative importance of these impacts remains unclear, together they have contributed to statewide wetland loss rates of 115 km2/yr. Severe decreases in fisheries production, storm surge protection, and extensive damage to minerals extraction infrastructure are expected to result from the landscape change in coastal Louisiana, if it continues to occur unchecked for the next several decades. Climate change impacts are expected to exacerbate these problems.
Restoration efforts among state and federal governments, university institutions, and the private sector aim to provide a modeling framework that can guide restoration decisions to reduce wetland loss. These models simulate variability in vegetation community structure and production which is driven by variability in salinity and wetland inundation. However, comprehensive, long-term field datasets that are necessary to determine how marsh vegetation community composition and productivity respond to salinity and inundation are not available. As a result, the thresholds defining habitat switching and the parameters relating productivity to hydrology are currently based on very limited data, mostly from laboratory experiments, or consensus obtained from the best professional judgment of multiple scientists.
Vegetation production and decomposition data are critical to understand the contribution of organic matter to soil development and marsh elevation change. Land building models typically focus only on mineral sediment inputs, and to appropriately capture soil development, both above-ground and below-ground processes need to be quantified along with mineral sedimentation. Furthermore, to accurately predict changes in carbon storage capacity as a result of climate change, it is critical to understand how ecological functions such as primary production and decomposition vary across different wetland types.
Methodology for Addressing the Issue: We will employ a space for time substitution to show long-term effects of rising sea-level and increasing salinity on vegetation community structure, primary production and decomposition. Productivity and decomposition rates will be estimated for four wetland plant community types defined by salinity zones and dominant plant species. Wetland community types will include fresh marshes dominated by Panicum hemitomon (salinity: 0-0.5 ppt), intermediate marshes dominated by Sagittaria lancifolia (salinity: 0.5-5 ppt), brackish marshes dominated by Spartina patens (salinity: 5-12 ppt), and saline marshes dominated by Spartina alterniflora (salinity: 12-30 ppt). Because these wetland community types are primarily defined by their salinity zone of tolerance, treatment effects reflect the indirect effect of changing vegetation community structure on ecological function. For each wetland community, six sites will be established across two hydrologic basins (Figure 1). The experimental design is a randomized complete block design with sampling, where the hydrologic basins represent the block effect and wetland community types represent the main treatment effect, and plot-level measurements within each site represent sampling error.
Future Steps: Future studies will examine how warming and elevated CO2 will affect decomposition of plants across the landscape scale gradient, thus allowing us to better predict the fate of carbon storage with climate change. We will also determine the relationships between field-validated estimates of primary production and decomposition, plant biochemistry, and imaging spectroscopy to improve predictive capabilities of ecological models that rely on process-based data. Additionally, the production and decomposition data collected from this study will support efforts to refine existing sediment cohort models.
This study will employ a space for time substitution to show long-term effects of rising sea-level and increasing salinity on vegetation community structure, primary production and decomposition. Productivity and decomposition rates will be estimated for four wetland plant community types defined by salinity zones and dominant plant species.
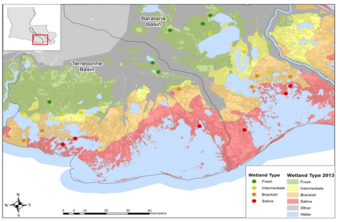
Science Issue and Relevance: Coastal areas comprise less than 20% of the total land area in the continental United States, yet they are home to over 50% of the nation’s population. High population densities in these regions often lead to severe environmental degradation as a result of flood control measures, commercial navigation support, and fossil fuels extraction. Effective restoration and management of these valuable coastal areas is of paramount importance, particularly in an age of changing climate.
Relative sea level rise rates have been observed in some regions of the northern Gulf of Mexico in excess of 10 mm/yr. Pulsed fluvial inputs which once nourished wetlands of the Mississippi River delta plain have been virtually eliminated for nearly a century because of the hydrologic separation of containment levees. Impoundment effects resulting from the construction of oil and gas pipeline canals and spoil banks have altered flooding regimes, and deep channel access has increased tidal energy and salinity through enhanced estuary-ocean exchange. Although the relative importance of these impacts remains unclear, together they have contributed to statewide wetland loss rates of 115 km2/yr. Severe decreases in fisheries production, storm surge protection, and extensive damage to minerals extraction infrastructure are expected to result from the landscape change in coastal Louisiana, if it continues to occur unchecked for the next several decades. Climate change impacts are expected to exacerbate these problems.
Restoration efforts among state and federal governments, university institutions, and the private sector aim to provide a modeling framework that can guide restoration decisions to reduce wetland loss. These models simulate variability in vegetation community structure and production which is driven by variability in salinity and wetland inundation. However, comprehensive, long-term field datasets that are necessary to determine how marsh vegetation community composition and productivity respond to salinity and inundation are not available. As a result, the thresholds defining habitat switching and the parameters relating productivity to hydrology are currently based on very limited data, mostly from laboratory experiments, or consensus obtained from the best professional judgment of multiple scientists.
Vegetation production and decomposition data are critical to understand the contribution of organic matter to soil development and marsh elevation change. Land building models typically focus only on mineral sediment inputs, and to appropriately capture soil development, both above-ground and below-ground processes need to be quantified along with mineral sedimentation. Furthermore, to accurately predict changes in carbon storage capacity as a result of climate change, it is critical to understand how ecological functions such as primary production and decomposition vary across different wetland types.
Methodology for Addressing the Issue: We will employ a space for time substitution to show long-term effects of rising sea-level and increasing salinity on vegetation community structure, primary production and decomposition. Productivity and decomposition rates will be estimated for four wetland plant community types defined by salinity zones and dominant plant species. Wetland community types will include fresh marshes dominated by Panicum hemitomon (salinity: 0-0.5 ppt), intermediate marshes dominated by Sagittaria lancifolia (salinity: 0.5-5 ppt), brackish marshes dominated by Spartina patens (salinity: 5-12 ppt), and saline marshes dominated by Spartina alterniflora (salinity: 12-30 ppt). Because these wetland community types are primarily defined by their salinity zone of tolerance, treatment effects reflect the indirect effect of changing vegetation community structure on ecological function. For each wetland community, six sites will be established across two hydrologic basins (Figure 1). The experimental design is a randomized complete block design with sampling, where the hydrologic basins represent the block effect and wetland community types represent the main treatment effect, and plot-level measurements within each site represent sampling error.
Future Steps: Future studies will examine how warming and elevated CO2 will affect decomposition of plants across the landscape scale gradient, thus allowing us to better predict the fate of carbon storage with climate change. We will also determine the relationships between field-validated estimates of primary production and decomposition, plant biochemistry, and imaging spectroscopy to improve predictive capabilities of ecological models that rely on process-based data. Additionally, the production and decomposition data collected from this study will support efforts to refine existing sediment cohort models.