Release Date: MAY 29, 2020
Residents in California, Oregon, and Washington have been told that earthquake early warning (EEW) is at their doorstep and will alert them to the shaking from an earthquake before it arrives. Some media reports have said there will be “up to a minute of warning”. The problem is, for onshore earthquakes these claims are exaggerated, and under some conditions a warning is not scientifically or technically possible. One USGS scientist has sorted through the challenges, the claims, and the data to determine what the possibilities and limitations really are for EEW, and the results may surprise you.
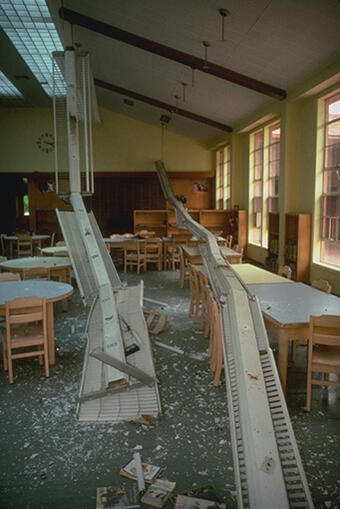
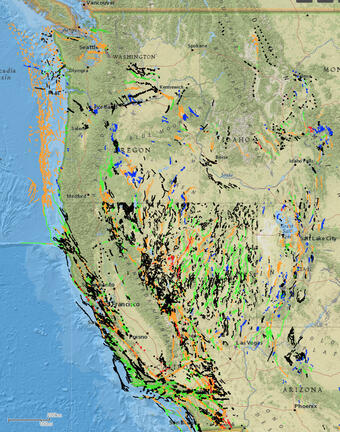
The premise behind EEW is that when an earthquake starts, the closest stations will register the fastest seismic waves, the P-waves, and from that information the impact of later-arriving, larger S-waves can be estimated and distributed to systems and people who may be in their path before the potentially damaging shaking arrives. Because radio waves travel much faster than seismic waves, it may be possible for the warnings to precede the arrival of the earthquake shaking. The process involves (1) determining how the earthquake will evolve, that is, how large will it probably get, based on the initial nature of the P-waves, (2) sending that information to a distribution system, and (3) transmitting the information to a large number of receivers (people or systems), who then have time to take an action based on the information. The goal of EEW is to “reduce harm or impacts of earthquakes” by providing warnings in sufficient time to make a difference.
A crucial piece of information that could be better communicated is that EEW alerts typically have a blind zone, an area around the earthquake epicenter that cannot be alerted before the strong shaking starts. The blind zone exists because each step in the process takes a bit of time–time during which the strong earthquake shaking may have already begun to impact the areas closest to the earthquake epicenter, the areas that will be shaken the strongest. A previous paper by another USGS scientist looked at the first step in the process, the estimation of the magnitude as the earthquake starts and continues to happen. Based on the time it takes to determine that the earthquake could have a large enough magnitude to cause significant shaking, she concluded that for crustal/onshore earthquakes the lightest shaken areas will typically receive about 10+ seconds of warning, the moderately shaken areas will receive <10 seconds, and the areas with damaging shaking will typically receive little to no warning (that means they’re in the blind zone). The most recent study described here builds on that conclusion, noting the additional delays not considered in earlier studies; these additional delays involve communicating the alert and responding to an alert. The cumulative delays in receiving and acting on an early warning enlarge the blind zone so that it encompasses not only the strongest shaken areas, but some or most of the moderately shaken areas too.
Based on data from the Japan EEW, the fastest alerting through public communications takes about 2-3 seconds. Given this communication latency Wald notes that “current seismic network and communication limitations result in about a 25-km (15-mi) radius zone centered on the epicenter for which alerts are not possible.” And, currently, a 2-3 second communication latency for cell phones is not achievable in the U.S.
Let’s look at some early warning systems that have been around for a while in other parts of the world before returning to the U.S. EEW is new to the U.S., but it’s been around for quite a long time in other parts of the world such as Mexico City and Japan. Japan has the longest-running EEW system in the world, so let’s take a look at how it’s been working. Japan has three different types of earthquakes: 1) distant, offshore subduction events, 2) deep events from bending of the subducting plate, and 3) onshore or just-offshore shallow crustal events.
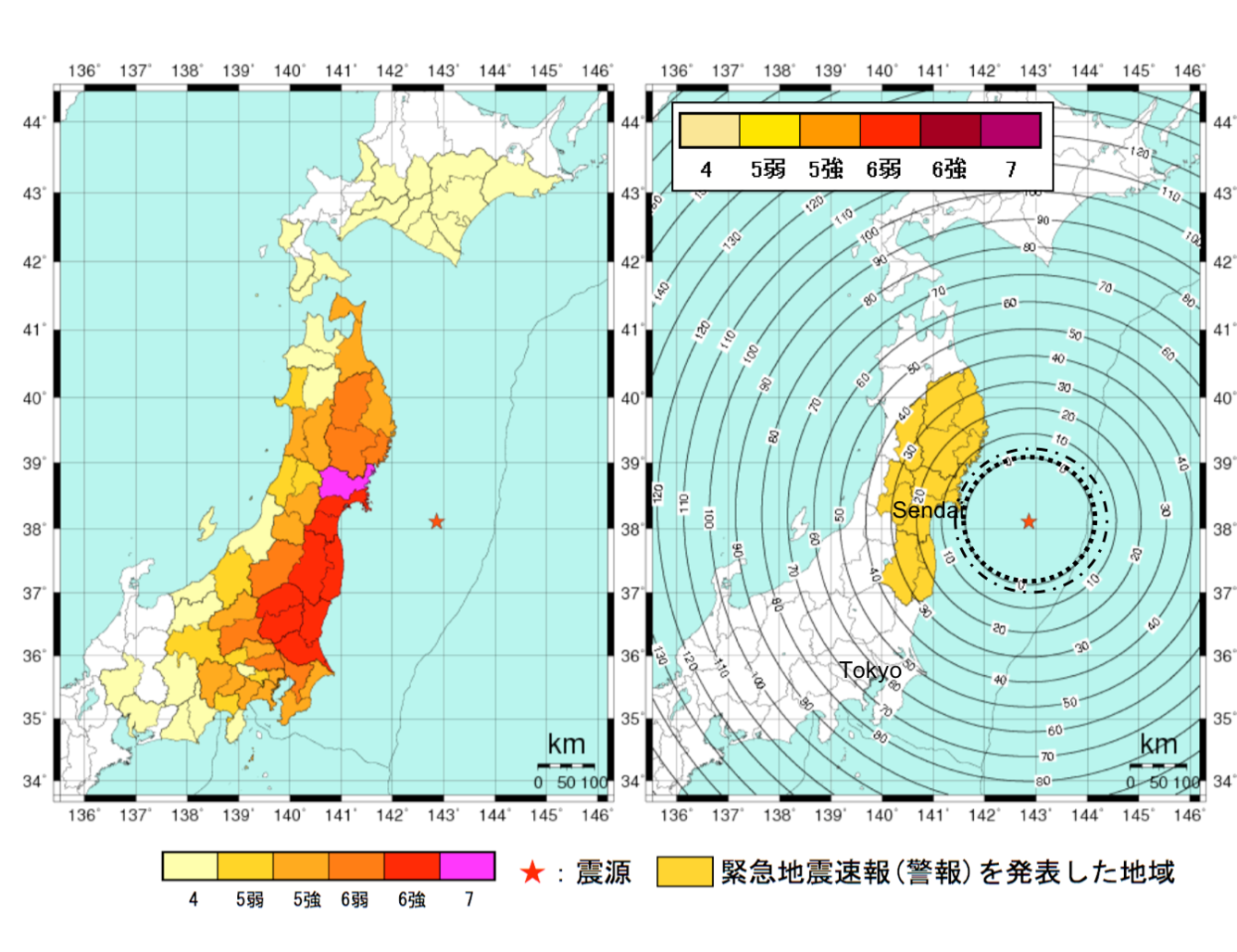
The M9.0 Tohoku-oki earthquake on March 11, 2011 was a distant off-shore subduction event. The map of estimated shaking and warning times ahead of the shaking shows that residents on the east coast had up to 15 seconds of warning while residents in more distant Tokyo, where shaking was less extreme but still “strong,” would have received up to a minute of warning had there not been other technical limitations in the performance of the system. The M6.7 Hokkaido earthquake on September 6, 2018 and the M7.0 Kumamoto earthquake on April 16, 2018 are examples of on-shore shallow crustal earthquakes. The map for each shows that in the areas of greatest intensity shaking there was no warning; they were in the blind zone. These examples show, according to Wald, that even the “best EEW system in the world cannot provide adequate warning where it is needed the most – (in) the blind zone.”
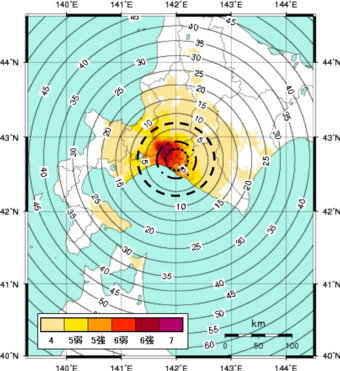
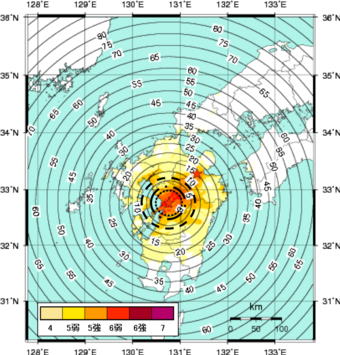
To see what the blind zone may look like for California earthquakes with the additional delays due to warning communication and response, let’s look at how EEW would have worked for the 1994 M6.7 Northridge, CA earthquake that caused 40 billion dollars in damage, several dozen fatalities, and thousands of injuries. The inner-most circle around the epicenter shows the alert time, the time it takes to determine a warning should be sent; the next circle moving out from the epicenter is the warning time, the time users will receive a notification based on the 2-second communication latency; the 3rd largest circle is the response time, the time when people would respond, based on an average of 5 seconds for users to react, as indicated in social science literature.
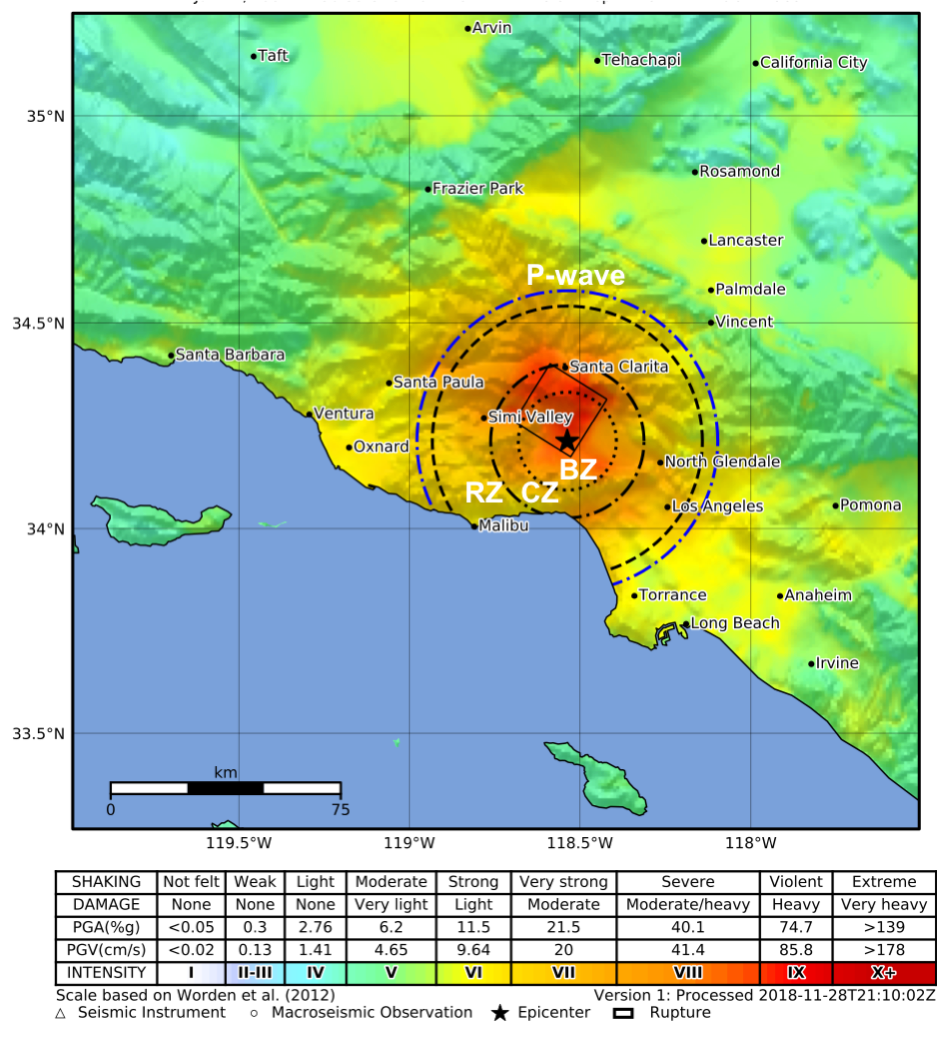
Even without the latencies for communication and reaction, those in the most strongly shaken area would not have received a warning before the shaking started, and the more lightly shaken areas with little damage would have received only a few seconds of warning. With all three latencies, only the very lightest shaken areas would have received a warning. The areas that were shaken severely and had the most damage, injuries and fatalities would have received no early warning or perhaps late ones. Injuries and fatalities mostly occur in areas with MMI’s of VII or higher, areas typically inside the blind zone. A more recent event, the July 2019 M6.4 Ridgecrest, CA earthquake occurred after the ShakeAlertÒ system was in operation. The Ridgecrest area with the most damage was in the blind zone and received no warning.
According to Wald: “It is fundamental to communicate these limitations–that substantive warning times occur primarily at relatively low shaking levels–particularly when promoting EEW through the media.” Almost all deadly California earthquakes have occurred inland, like Northridge, where those areas most strongly shaken are in the blind zone.
Fortunately, an offshore Cascadian subduction zone event in the Pacific Northwest would be more analogous to the offshore Japan events. However, the U.S. does not yet have offshore sensors for early detection of an offshore earthquake , so many seconds of early warning would be lost waiting for the shaking to reach the onshore seismic sensors, although some useful warning could be provided to inland cities such as Seattle and Portland. (Note that there are efforts underway to hopefully add earthquake sensors to the offshore environment in the future, thereby enhancing warning times).
What about automated systems? Bay Area Rapid Transit (BART) is an early adopter of the ShakeAlert® EEW system, and yet it takes about 10 seconds to slow a BART train from 70 to 40 mph. The warning time in strongly shaken areas are likely to be <10 seconds (as explained above), so the ability to stop a train that’s in an area with severe shaking is not likely to be a realistic expectation. Nevertheless, slowing trains may be possible and potentially beneficial. Other automated systems may have shorter reaction times but will still have very little to no warning in the most severely shaken areas in the blind zone.
Despite the often overstated warning times from the media (e.g., “up to a minute”) for earthquakes on the northern and southern San Andreas Fault, the reality is for most areas of strong shaking, warning times are very short or non-existent. This reality needs to be communicated to the public and other potential users of EEW so that the expectations are aligned with what the system is actually able to deliver.
Note: Quotes are from the publication summarized in this article, referenced below.
- written by Lisa Wald, U.S. Geological Survey, May 2020
For More Information
-
Wald, D. J. (2020). Practical limitations of earthquake early warning. Earthquake Spectra.
- Earthquake Early Warning Research
The Scientist Behind the Science
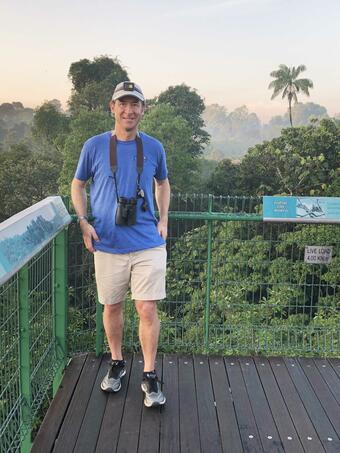
David Wald’s research aims to provide information for the mitigation of earthquake damage and losses, including information about shaking intensity and estimated impacts immediately after an earthquake to aid in the response. He led the creation of the Did You Feel It?, ShakeMap, ShakeCast, and PAGER(2) applications and online tools for this purpose. In his free time, he likes to run and bike ride in the mountains, and he also enjoys birdwatching.
Release Date: MAY 29, 2020
Residents in California, Oregon, and Washington have been told that earthquake early warning (EEW) is at their doorstep and will alert them to the shaking from an earthquake before it arrives. Some media reports have said there will be “up to a minute of warning”. The problem is, for onshore earthquakes these claims are exaggerated, and under some conditions a warning is not scientifically or technically possible. One USGS scientist has sorted through the challenges, the claims, and the data to determine what the possibilities and limitations really are for EEW, and the results may surprise you.
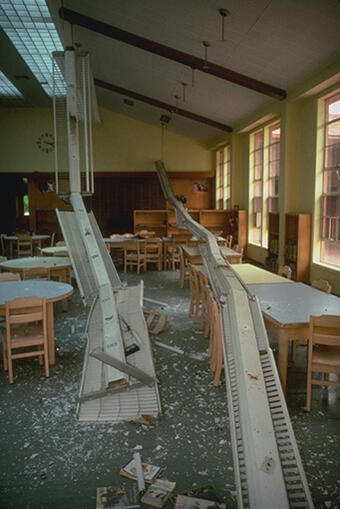
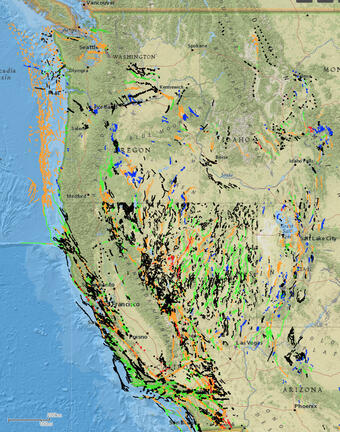
The premise behind EEW is that when an earthquake starts, the closest stations will register the fastest seismic waves, the P-waves, and from that information the impact of later-arriving, larger S-waves can be estimated and distributed to systems and people who may be in their path before the potentially damaging shaking arrives. Because radio waves travel much faster than seismic waves, it may be possible for the warnings to precede the arrival of the earthquake shaking. The process involves (1) determining how the earthquake will evolve, that is, how large will it probably get, based on the initial nature of the P-waves, (2) sending that information to a distribution system, and (3) transmitting the information to a large number of receivers (people or systems), who then have time to take an action based on the information. The goal of EEW is to “reduce harm or impacts of earthquakes” by providing warnings in sufficient time to make a difference.
A crucial piece of information that could be better communicated is that EEW alerts typically have a blind zone, an area around the earthquake epicenter that cannot be alerted before the strong shaking starts. The blind zone exists because each step in the process takes a bit of time–time during which the strong earthquake shaking may have already begun to impact the areas closest to the earthquake epicenter, the areas that will be shaken the strongest. A previous paper by another USGS scientist looked at the first step in the process, the estimation of the magnitude as the earthquake starts and continues to happen. Based on the time it takes to determine that the earthquake could have a large enough magnitude to cause significant shaking, she concluded that for crustal/onshore earthquakes the lightest shaken areas will typically receive about 10+ seconds of warning, the moderately shaken areas will receive <10 seconds, and the areas with damaging shaking will typically receive little to no warning (that means they’re in the blind zone). The most recent study described here builds on that conclusion, noting the additional delays not considered in earlier studies; these additional delays involve communicating the alert and responding to an alert. The cumulative delays in receiving and acting on an early warning enlarge the blind zone so that it encompasses not only the strongest shaken areas, but some or most of the moderately shaken areas too.
Based on data from the Japan EEW, the fastest alerting through public communications takes about 2-3 seconds. Given this communication latency Wald notes that “current seismic network and communication limitations result in about a 25-km (15-mi) radius zone centered on the epicenter for which alerts are not possible.” And, currently, a 2-3 second communication latency for cell phones is not achievable in the U.S.
Let’s look at some early warning systems that have been around for a while in other parts of the world before returning to the U.S. EEW is new to the U.S., but it’s been around for quite a long time in other parts of the world such as Mexico City and Japan. Japan has the longest-running EEW system in the world, so let’s take a look at how it’s been working. Japan has three different types of earthquakes: 1) distant, offshore subduction events, 2) deep events from bending of the subducting plate, and 3) onshore or just-offshore shallow crustal events.
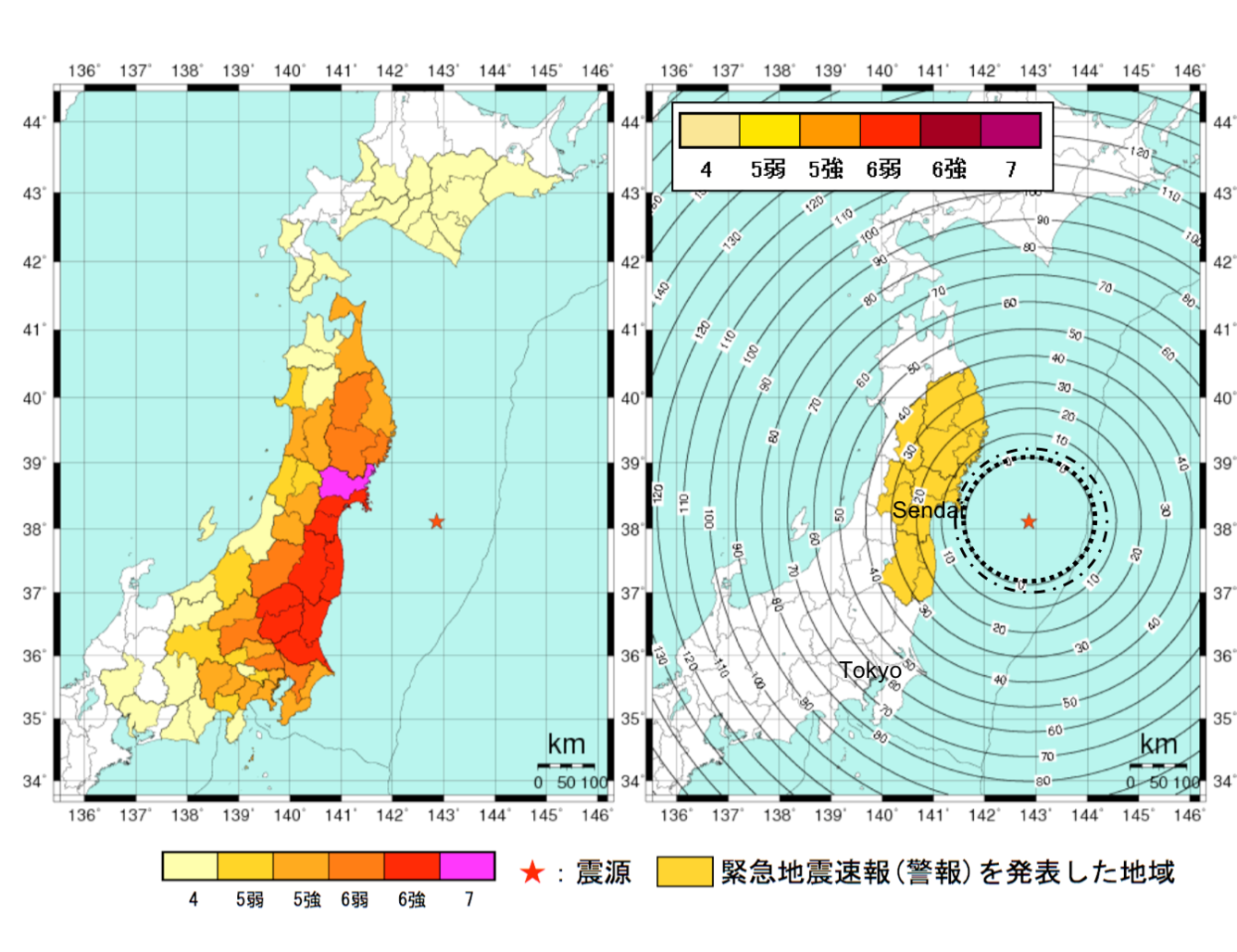
The M9.0 Tohoku-oki earthquake on March 11, 2011 was a distant off-shore subduction event. The map of estimated shaking and warning times ahead of the shaking shows that residents on the east coast had up to 15 seconds of warning while residents in more distant Tokyo, where shaking was less extreme but still “strong,” would have received up to a minute of warning had there not been other technical limitations in the performance of the system. The M6.7 Hokkaido earthquake on September 6, 2018 and the M7.0 Kumamoto earthquake on April 16, 2018 are examples of on-shore shallow crustal earthquakes. The map for each shows that in the areas of greatest intensity shaking there was no warning; they were in the blind zone. These examples show, according to Wald, that even the “best EEW system in the world cannot provide adequate warning where it is needed the most – (in) the blind zone.”
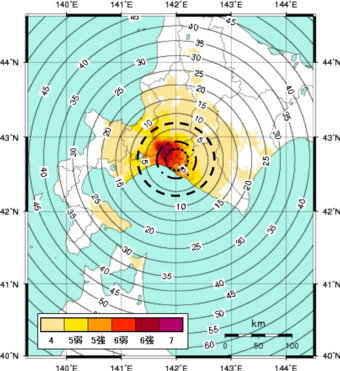
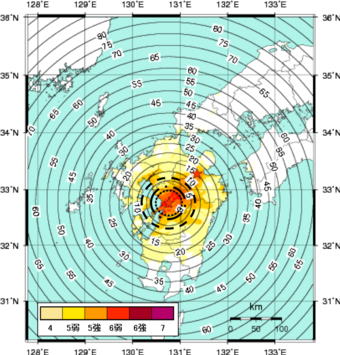
To see what the blind zone may look like for California earthquakes with the additional delays due to warning communication and response, let’s look at how EEW would have worked for the 1994 M6.7 Northridge, CA earthquake that caused 40 billion dollars in damage, several dozen fatalities, and thousands of injuries. The inner-most circle around the epicenter shows the alert time, the time it takes to determine a warning should be sent; the next circle moving out from the epicenter is the warning time, the time users will receive a notification based on the 2-second communication latency; the 3rd largest circle is the response time, the time when people would respond, based on an average of 5 seconds for users to react, as indicated in social science literature.
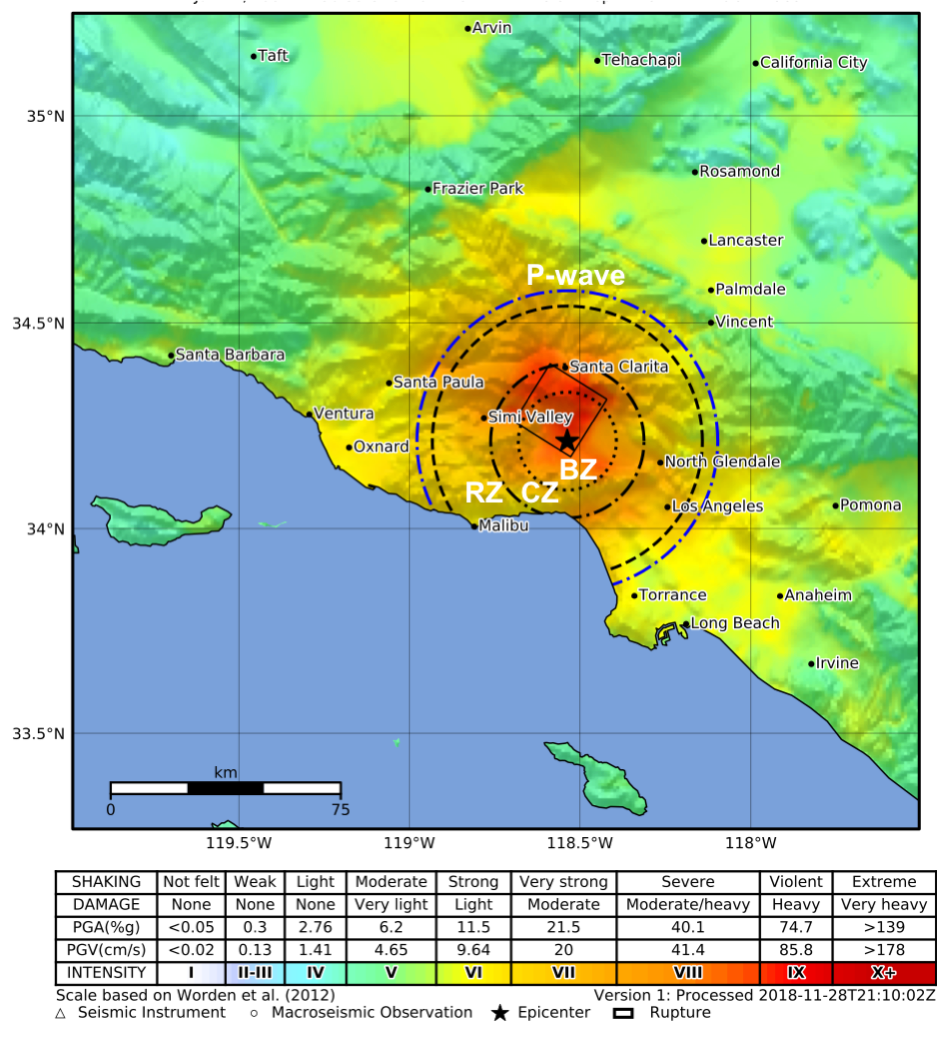
Even without the latencies for communication and reaction, those in the most strongly shaken area would not have received a warning before the shaking started, and the more lightly shaken areas with little damage would have received only a few seconds of warning. With all three latencies, only the very lightest shaken areas would have received a warning. The areas that were shaken severely and had the most damage, injuries and fatalities would have received no early warning or perhaps late ones. Injuries and fatalities mostly occur in areas with MMI’s of VII or higher, areas typically inside the blind zone. A more recent event, the July 2019 M6.4 Ridgecrest, CA earthquake occurred after the ShakeAlertÒ system was in operation. The Ridgecrest area with the most damage was in the blind zone and received no warning.
According to Wald: “It is fundamental to communicate these limitations–that substantive warning times occur primarily at relatively low shaking levels–particularly when promoting EEW through the media.” Almost all deadly California earthquakes have occurred inland, like Northridge, where those areas most strongly shaken are in the blind zone.
Fortunately, an offshore Cascadian subduction zone event in the Pacific Northwest would be more analogous to the offshore Japan events. However, the U.S. does not yet have offshore sensors for early detection of an offshore earthquake , so many seconds of early warning would be lost waiting for the shaking to reach the onshore seismic sensors, although some useful warning could be provided to inland cities such as Seattle and Portland. (Note that there are efforts underway to hopefully add earthquake sensors to the offshore environment in the future, thereby enhancing warning times).
What about automated systems? Bay Area Rapid Transit (BART) is an early adopter of the ShakeAlert® EEW system, and yet it takes about 10 seconds to slow a BART train from 70 to 40 mph. The warning time in strongly shaken areas are likely to be <10 seconds (as explained above), so the ability to stop a train that’s in an area with severe shaking is not likely to be a realistic expectation. Nevertheless, slowing trains may be possible and potentially beneficial. Other automated systems may have shorter reaction times but will still have very little to no warning in the most severely shaken areas in the blind zone.
Despite the often overstated warning times from the media (e.g., “up to a minute”) for earthquakes on the northern and southern San Andreas Fault, the reality is for most areas of strong shaking, warning times are very short or non-existent. This reality needs to be communicated to the public and other potential users of EEW so that the expectations are aligned with what the system is actually able to deliver.
Note: Quotes are from the publication summarized in this article, referenced below.
- written by Lisa Wald, U.S. Geological Survey, May 2020
For More Information
-
Wald, D. J. (2020). Practical limitations of earthquake early warning. Earthquake Spectra.
- Earthquake Early Warning Research
The Scientist Behind the Science
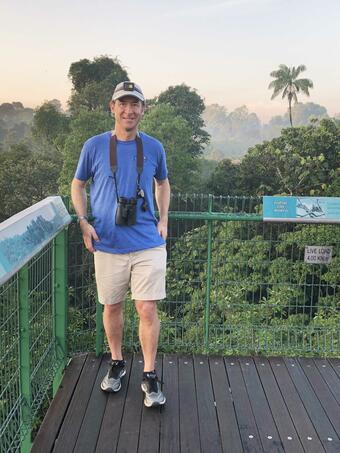
David Wald’s research aims to provide information for the mitigation of earthquake damage and losses, including information about shaking intensity and estimated impacts immediately after an earthquake to aid in the response. He led the creation of the Did You Feel It?, ShakeMap, ShakeCast, and PAGER(2) applications and online tools for this purpose. In his free time, he likes to run and bike ride in the mountains, and he also enjoys birdwatching.