The soil core (top) was collected from Bass Creek, Yarmouth, MA, which was restored in 2008. From this soil core, scientists recreated the elevation of the marsh surface over the past 100 years, as well as how quickly elevation changed.
Sea level Rise and Carbon Cycle Processes in Managed Coastal Wetlands
The U.S. Geological Survey is working to inform decision makers at Federal land management agencies, including the U.S. Fish and Wildlife Service, the National Park Service, state government agencies and private entities, on the role of coastal wetlands in climate change mitigation and adaptation. This includes assessing opportunities for ecosystem restoration to enhance soil carbon sequestration, reduce methane emissions, and to increase carbon and alkalinity export to the coastal ocean. This comprehensive knowledge of carbon cycle processes is necessary to support the integration of coastal ecosystems within programs that reduce and inventory greenhouse gas emissions, including development of programs for greenhouse gas monitoring, reporting, and verification (MRV).
In this project, USGS scientists are studying how human modifications of coastal hydrology affect wetland resilience, migration, hazard mitigation, ecosystem condition, and carbon cycle processes. This knowledge will help guide decisions made by federal, state, and private land management organizations.
Why is this work important?
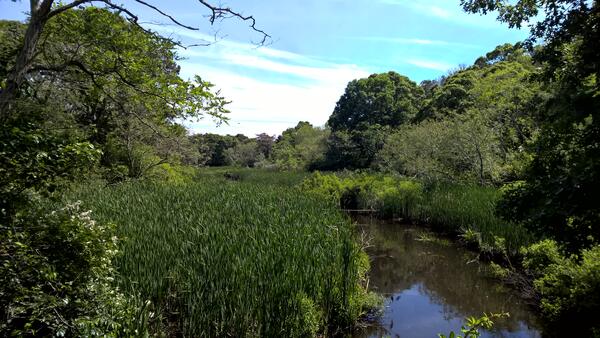
Wetlands can capture large quantities of carbon dioxide from the atmosphere and store it in their soil and plants—a process known as carbon sequestration. In fact, they are such significant carbon sinks that they can store carbon that has accumulated over hundreds to thousands of years.
Persistence of these habitats in the 21st century depends on relatively near-term decisions regarding transportation infrastructure and other responses by society to sea level rise hazards. Maintenance and new deployments of roads and coastal defense structures, such as seawalls and dikes, can interfere with coastal hydrology, causing drainage or impoundment, and prevent tidal wetlands from migrating in response to sea level rise. When coastal wetlands are drained, impounded, or restricted, it jeopardizes the quality and existence of these vital ecosystems.
For centuries, in the U.S. and globally, there has been ongoing deployment of human-built structures that change coastal hydrology, including dikes, berms, levees, ditches, and seawalls. Additionally, transportation networks, including roads and railroads, cause widespread incidental tidal restriction in coastal wetlands. In a first set of national-scale analysis, we have estimated that more than 2.5 million acres of tidal wetlands in the conterminous United States are impounded, tidally-restricted, or drained behind infrastructure. In response to climate change and the accelerating rate of sea level rise, deployment of dikes is expected to increase in the coming decades, with potential for substantial additional impact on wetland ecological condition, on their ability to migrate landward in response to sea level rise, and ultimately the persistence of these vital ecosystems.
Coastal wetlands and mangroves that have been damaged or destroyed can lose their carbon sequestration capacity and the large quantities of carbon stored there can be released into the atmosphere. Improved management of salt marshes and mangroves, leading to enhanced conservation and restoration, is therefore a crucial climate change mitigation strategy—providing both enhanced carbon dioxide removal and avoided methane emissions.
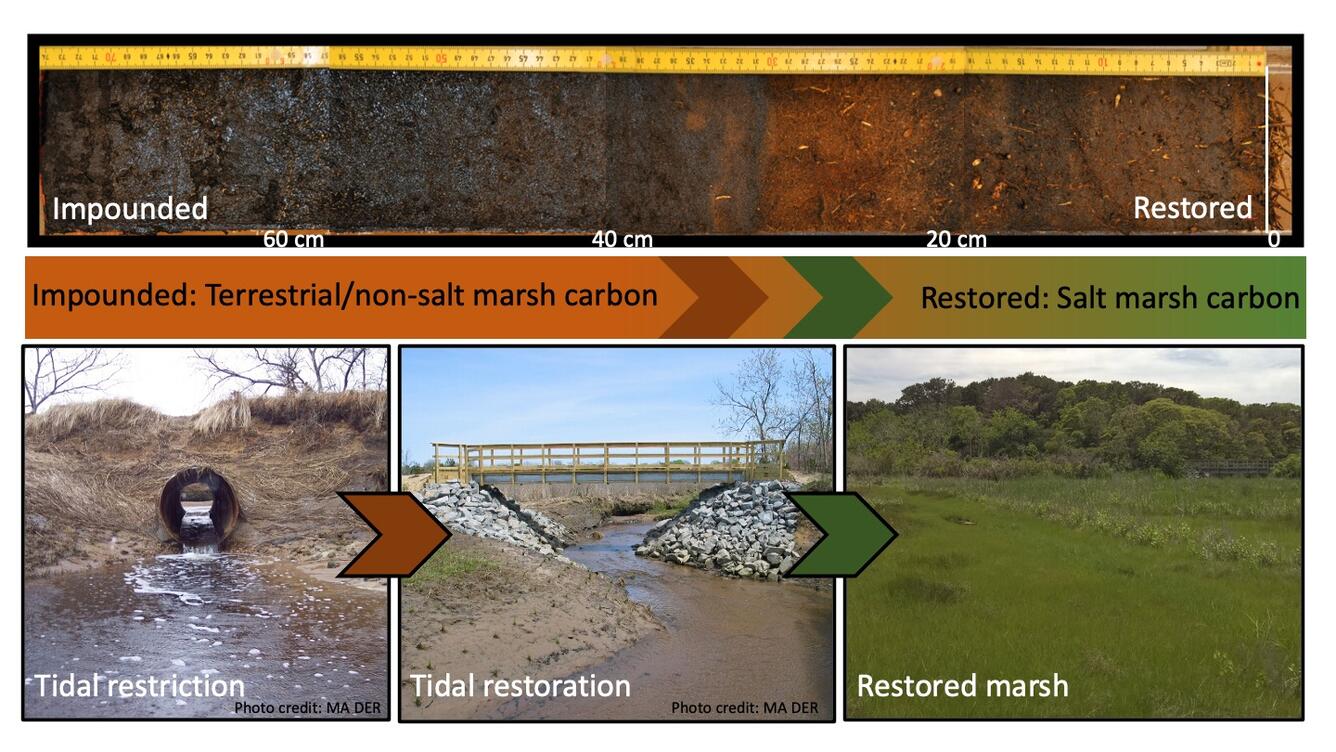
What we do?
The USGS and colleagues will develop functional maps, models and predictions for the existing and future intertidal landscape. Specific tasks include:
- As a part of the NPS Herring River restoration project in the Cape Cod National Seashore, scientists are analyzing the blue carbon value of dike removal and wetland restoration. They are creating site scale models to predict groundwater and surface water hydrological changes in response to sea level rise, biogeochemical and vegetation responses that drive soil elevation change, changes in soil and vegetation carbon stocks, methane emissions and lateral export of carbon to the coastal ocean.
Read more about the project!
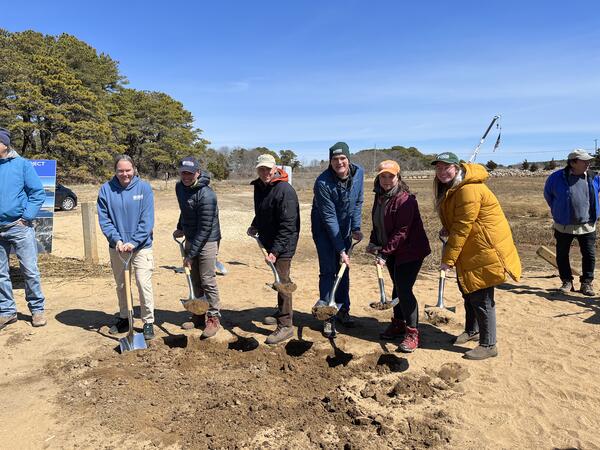
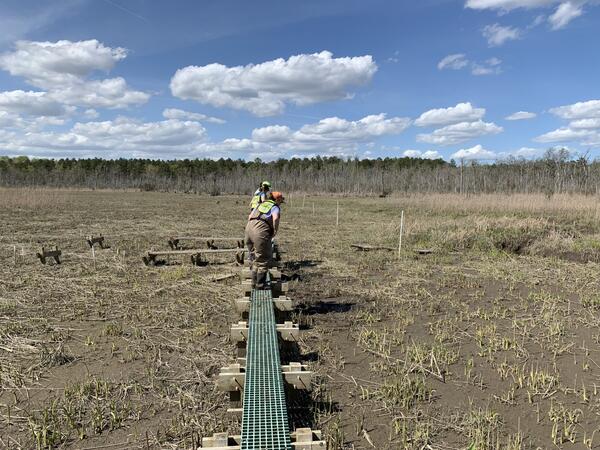
- Mapping wetland management conditions, the suite of structures that interact with coastal wetlands and hydrology. Management condition has not been sufficiently mapped in existing land cover databases which has limited utility to guide blue carbon implementation. These new products will identify degraded wetlands, and opportunities for wetland restoration.
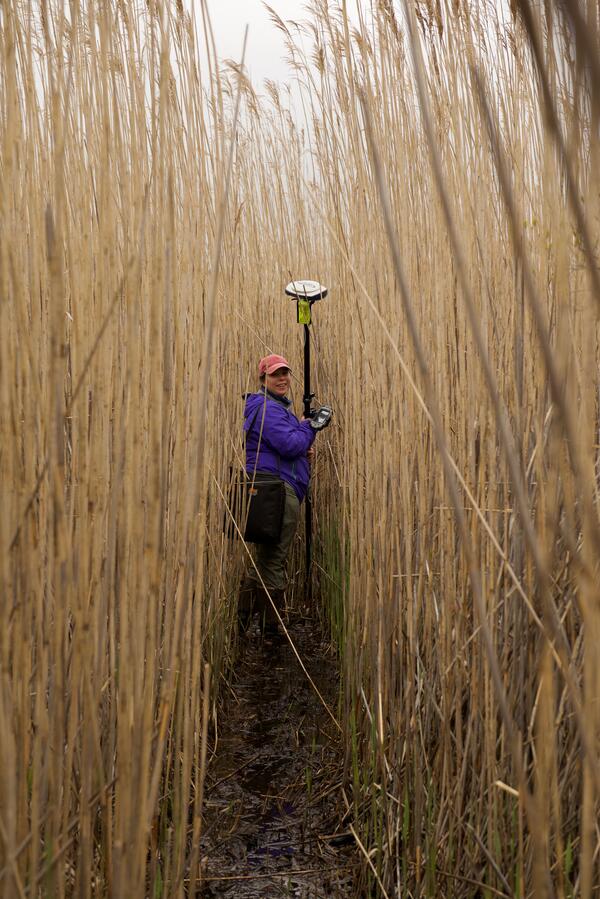
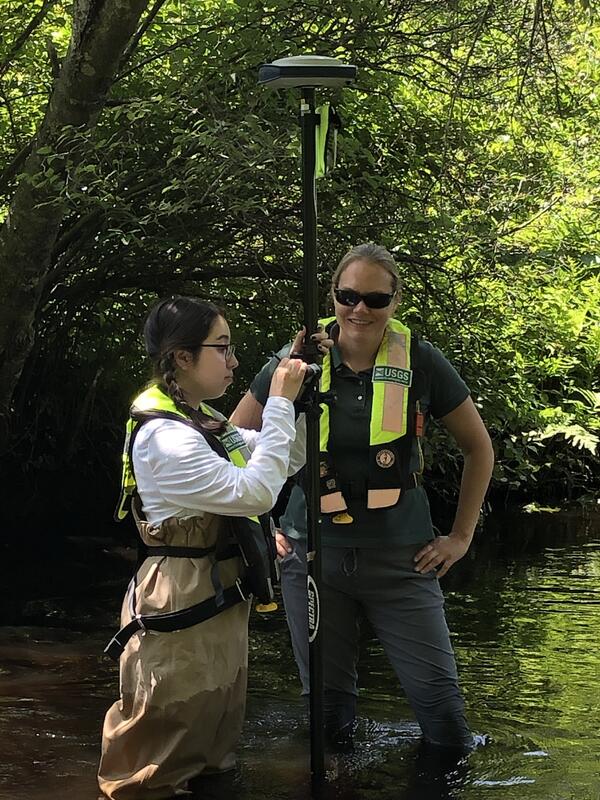
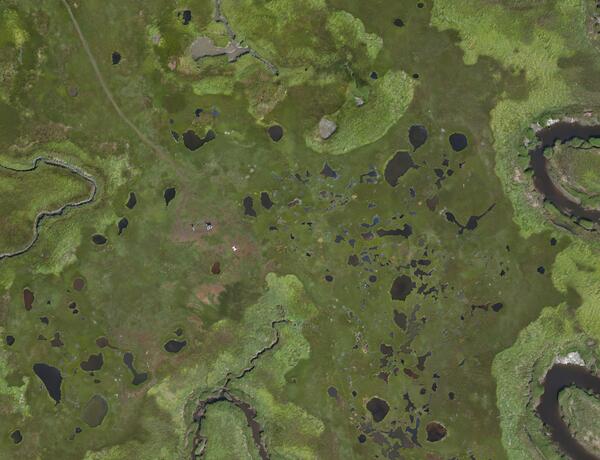
- Use time-series analysis of the full record of Landsat data (beginning in 1986) to detect hydrological condition and tidal connectivity in coastal wetlands, as well as monitor past and future changes in wetland distribution and hydrology.
- Develop national scale models to estimate the amount of carbon exported and alkalinity from tidal wetlands to the coastal ocean, and examine the potential for long-term, millennial-scale, storage in ocean water. Long term storage in seawater is an unrecognized pathway for carbon sequestration/carbon dioxide removal from the atmosphere (CDR). Model implementation will be supported by maps and satellite data to indicate the management condition of tidal wetlands, from local to national scales.
Read more about the project!
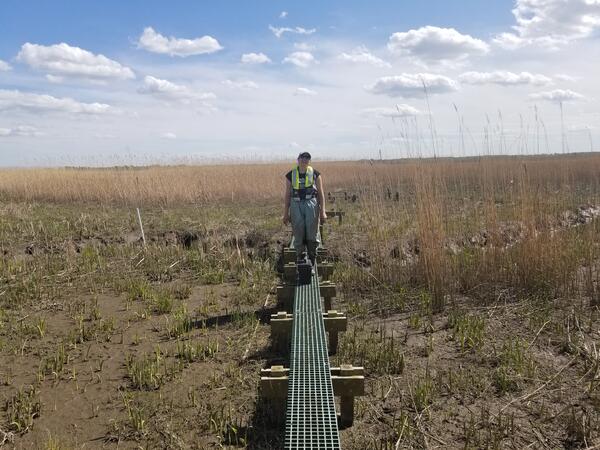
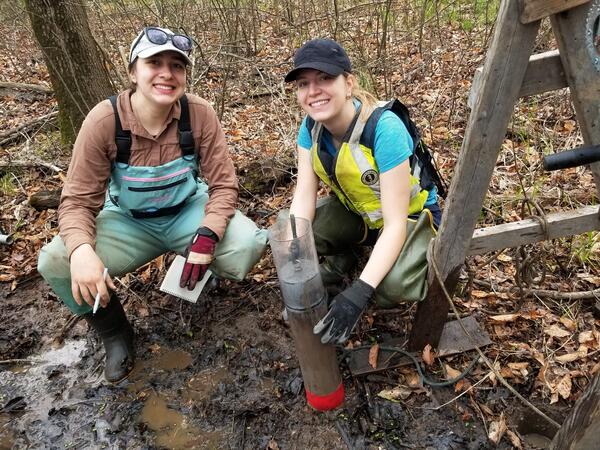
- Examine the potential for enhanced weathering of alkaline minerals in tidal wetlands. These alkaline minerals may be used as an approach to accelerate the export of dissolved carbon and alkalinity, with co-benefits including mitigation of ocean acidification, and providing an elevation subsidy for wetland soils.
Read more about the project!
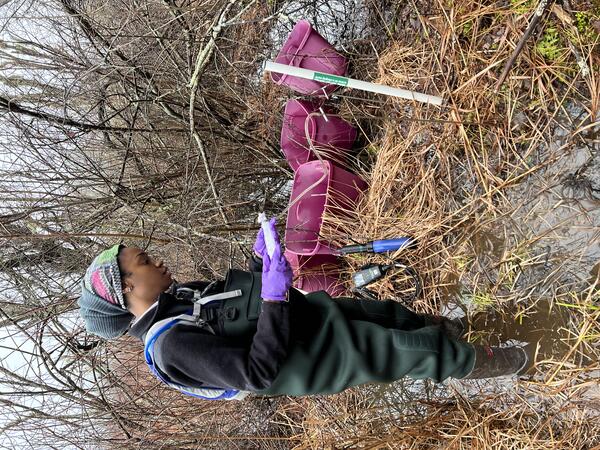
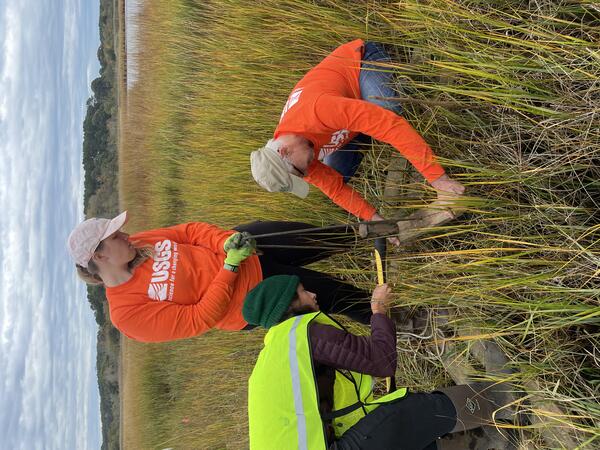
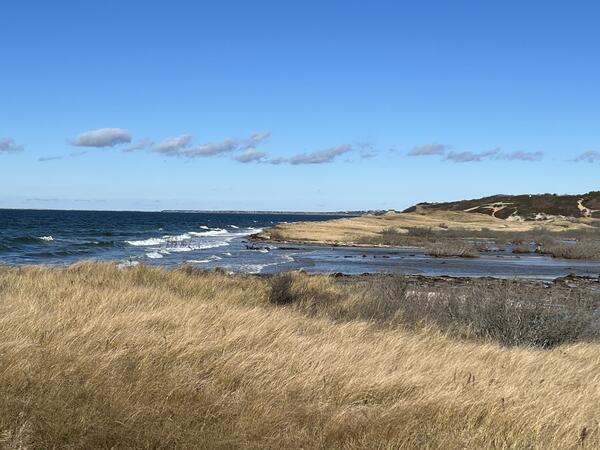
- Evaluate the biogeochemical changes that occur upon reintroduction of salt water to wetlands when historical tidal restriction has resulted in subsidence, acidified soils, and carbon loss. There is limited real world data to inform water quality consequences when there is rewetting of salt marsh peat with seawater. This water quality data can calibrate reactive transport models which inform goals and strategies for adaptive management plans such as water quality and impacts on shellfish beds.
Learn more about the project!
- Quantify environmental and geomorphic drivers of lateral carbon export to the ocean to inform numerical model development as well as provide data for coastal carbon budgets. Although not well known, tidal wetlands remove large amounts of carbon dioxide from the atmosphere, and export the carbon to the coastal ocean. Developing the first predictive model of carbon exports from marshes will provide a tool for predicting future changes in coastal carbon budgets with continued sea level rise.
More on the project!
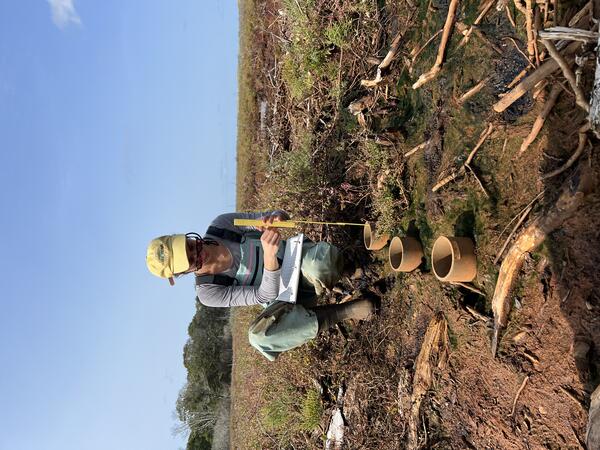
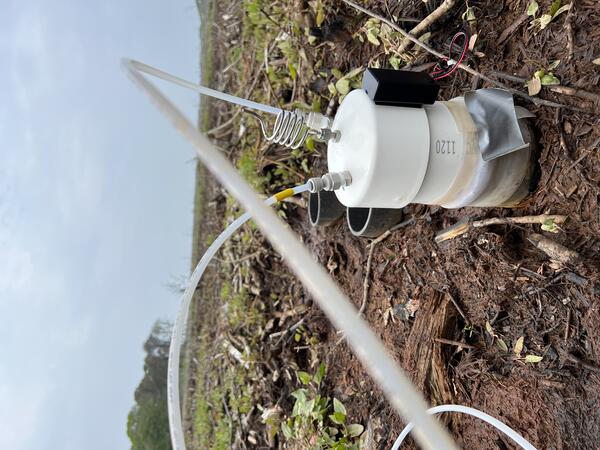
- Assess the net ecosystem carbon budget of wetlands in Louisiana. Wetlands are undergoing a variety of restoration and management actions with the goal to provide tools for managers to plan for future resiliency. As a part of the Deepwater Horizon oil spill restoration effort, the carbon capture of flora, fauna and soils associated with coastal wetland restoration will be evaluated to determine what methods create more resistant marshes with high rates of carbon sequestration.
More on the Gulf Spill Restoration Project.
- Explore drivers of coastal wetland accretion in the face of rising seas and human impacts to understand how burial of organic matter, sediment, and geomorphic evolution of salt marshes is tied to ongoing resilience and associated climate benefits.
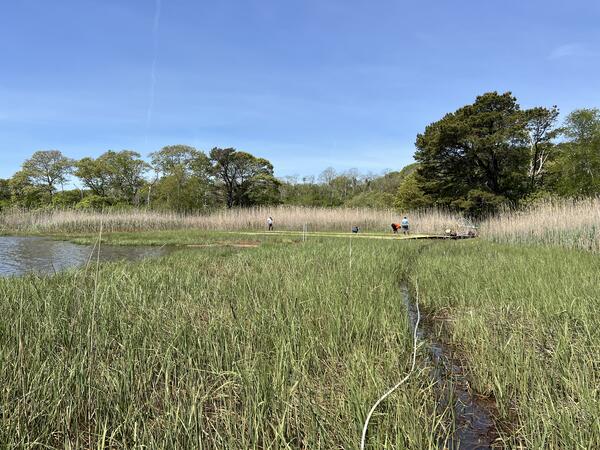
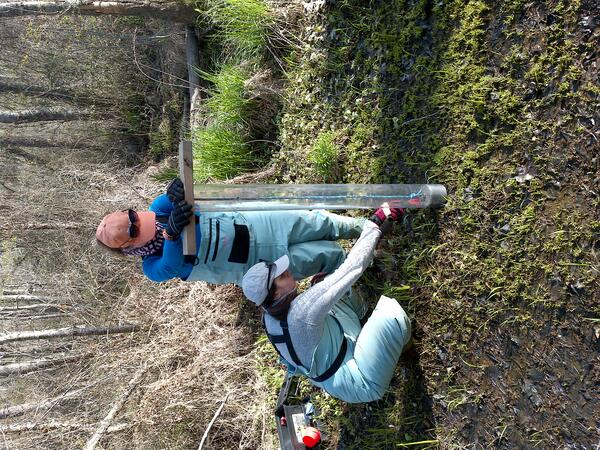
This work is being conducted for current, future, and potential intertidal space throughout the conterminous United States (CONUS) and will include site-, state-, and regional-level analyses.
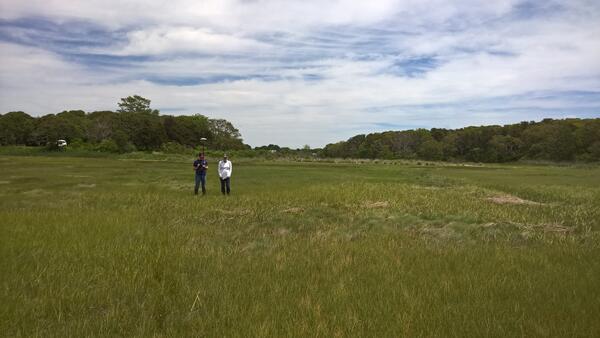
Partners, stakeholders, and end users for this project include the U.S. Fish and Wildlife Service, the National Park Service, state governments and private land managers, and non-governmental organizations. The USGS will continue to actively engage with them to ensure project objectives help meet their specific needs CONUS-wide.
Environmental Geochemistry
The soil core (top) was collected from Bass Creek, Yarmouth, MA, which was restored in 2008. From this soil core, scientists recreated the elevation of the marsh surface over the past 100 years, as well as how quickly elevation changed.
Carbon dioxide removal experiment led by Kevin Kroeger in Herring River in Wellfleet, Massachusetts.
Carbon dioxide removal experiment led by Kevin Kroeger in Herring River in Wellfleet, Massachusetts.
In collaboration with the National Park Service, Woods Hole Oceanographic Institution, and the Vesta Corporation, the Environmental Geosciences group researched a new approach to climate change mitigation. The team led an experiment at the Herring River site as a first examination.
In collaboration with the National Park Service, Woods Hole Oceanographic Institution, and the Vesta Corporation, the Environmental Geosciences group researched a new approach to climate change mitigation. The team led an experiment at the Herring River site as a first examination.
In collaboration with the National Park Service, Woods Hole Oceanographic Institution, and the Vesta Corporation, the Environmental Geosciences group researched a new approach to climate change mitigation. The team led an experiment at the Herring River site as a first examination.
In collaboration with the National Park Service, Woods Hole Oceanographic Institution, and the Vesta Corporation, the Environmental Geosciences group researched a new approach to climate change mitigation. The team led an experiment at the Herring River site as a first examination.
In collaboration with the National Park Service, Woods Hole Oceanographic Institution, and the Vesta Corporation, the Environmental Geosciences group researched a new approach to climate change mitigation. The team led an experiment at the Herring River site as a first examination.
In collaboration with the National Park Service, Woods Hole Oceanographic Institution, and the Vesta Corporation, the Environmental Geosciences group researched a new approach to climate change mitigation. The team led an experiment at the Herring River site as a first examination.
Image of a tidally restricted wetland in the Herring River Estuary, Cape Cod, MA
Image of a tidally restricted wetland in the Herring River Estuary, Cape Cod, MA
USGS samples water within wetlands to understand which environmental drivers impact carbon cycle and sediment accretion. Here USGS staff (Jennifer O'Keefe Suttles) pumps water from at Phragmites wetland, Mashpee, MA, to analyze at the Woods Hole Coastal and Marine Science Center Environmental Geochemistry lab.
USGS samples water within wetlands to understand which environmental drivers impact carbon cycle and sediment accretion. Here USGS staff (Jennifer O'Keefe Suttles) pumps water from at Phragmites wetland, Mashpee, MA, to analyze at the Woods Hole Coastal and Marine Science Center Environmental Geochemistry lab.
Wetlands play a key role in the coastal carbon cycle, removing carbon dioxide from the atmosphere during photosynthesis and releasing carbon dioxide and methane during respiration. The amount of methane emission is critical to determining how a wetland interacts with the global climate system.
Wetlands play a key role in the coastal carbon cycle, removing carbon dioxide from the atmosphere during photosynthesis and releasing carbon dioxide and methane during respiration. The amount of methane emission is critical to determining how a wetland interacts with the global climate system.
Instruments continue to collect vital data during workplace closures. Here staff perform maintenance on an eddy flux tower located within a phragmites wetland at the Cape Cod National Seashore. The instruments on this tower measure methane and CO2 fluxes related to plant and soil processes day in and day out for the entire year.
Instruments continue to collect vital data during workplace closures. Here staff perform maintenance on an eddy flux tower located within a phragmites wetland at the Cape Cod National Seashore. The instruments on this tower measure methane and CO2 fluxes related to plant and soil processes day in and day out for the entire year.
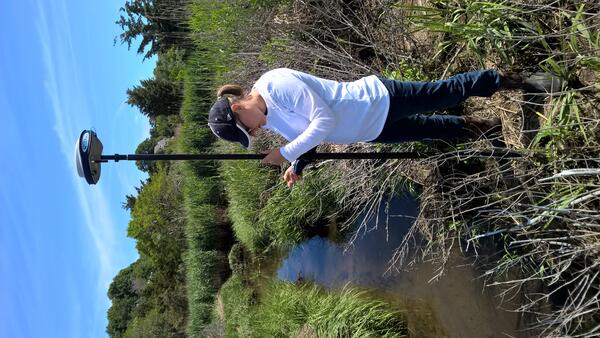
Meagan Eagle, USGS Research Scientist, collecting elevation points in Quivett Creek, Brewster, MA
linkMeagan Eagle, Research Scientists at the U.S. Geological Survey, collects an elevation point along the edge of Quivett Creek in Brewster, MA. This salt marsh was restored in 2005 by replacing a narrow culvert to allow full tidal flow once again.
Meagan Eagle, USGS Research Scientist, collecting elevation points in Quivett Creek, Brewster, MA
linkMeagan Eagle, Research Scientists at the U.S. Geological Survey, collects an elevation point along the edge of Quivett Creek in Brewster, MA. This salt marsh was restored in 2005 by replacing a narrow culvert to allow full tidal flow once again.
Two USGS scientists measure elevation at Bass Creek salt marsh, Yarmouth, MA.
Two USGS scientists measure elevation at Bass Creek salt marsh, Yarmouth, MA.
Salt marsh grass grows in the restored marsh at Bass Creek, Yarmouth, MA.
Salt marsh grass grows in the restored marsh at Bass Creek, Yarmouth, MA.
Carbonate chemistry and carbon sequestration driven by inorganic carbon outwelling from mangroves and saltmarshes
High-frequency variability of carbon dioxide fluxes in tidal water over a temperate salt marsh
Mapping methane reduction potential of tidal wetland restoration in the United States
Forecasting sea level rise-driven inundation in diked and tidally restricted coastal lowlands
Peat decomposition and erosion contribute to pond deepening in a temperate salt marsh
Mechanisms and magnitude of dissolved silica release from a New England salt marsh
Soil carbon consequences of historic hydrologic impairment and recent restoration in coastal wetlands
Impoundment increases methane emissions in Phragmites-invaded coastal wetlands
Detection and characterization of coastal tidal wetland change in the northeastern US using Landsat time series
Estimating the aboveground biomass and carbon stocks of tall shrubs in a prerestoration degraded salt marsh
Recent nitrogen storage and accumulation rates in mangrove soils exceed historic rates in the urbanized San Juan Bay Estuary (Puerto Rico, United States)
Recent carbon storage and burial exceed historic rates in the San Juan Bay estuary peri-urban mangrove forests (Puerto Rico, United States)
The U.S. Geological Survey is working to inform decision makers at Federal land management agencies, including the U.S. Fish and Wildlife Service, the National Park Service, state government agencies and private entities, on the role of coastal wetlands in climate change mitigation and adaptation. This includes assessing opportunities for ecosystem restoration to enhance soil carbon sequestration, reduce methane emissions, and to increase carbon and alkalinity export to the coastal ocean. This comprehensive knowledge of carbon cycle processes is necessary to support the integration of coastal ecosystems within programs that reduce and inventory greenhouse gas emissions, including development of programs for greenhouse gas monitoring, reporting, and verification (MRV).
In this project, USGS scientists are studying how human modifications of coastal hydrology affect wetland resilience, migration, hazard mitigation, ecosystem condition, and carbon cycle processes. This knowledge will help guide decisions made by federal, state, and private land management organizations.
Why is this work important?
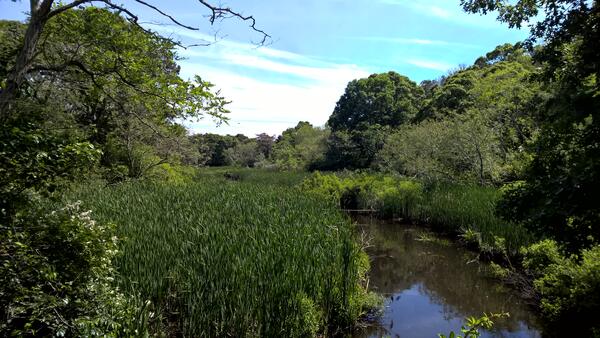
Wetlands can capture large quantities of carbon dioxide from the atmosphere and store it in their soil and plants—a process known as carbon sequestration. In fact, they are such significant carbon sinks that they can store carbon that has accumulated over hundreds to thousands of years.
Persistence of these habitats in the 21st century depends on relatively near-term decisions regarding transportation infrastructure and other responses by society to sea level rise hazards. Maintenance and new deployments of roads and coastal defense structures, such as seawalls and dikes, can interfere with coastal hydrology, causing drainage or impoundment, and prevent tidal wetlands from migrating in response to sea level rise. When coastal wetlands are drained, impounded, or restricted, it jeopardizes the quality and existence of these vital ecosystems.
For centuries, in the U.S. and globally, there has been ongoing deployment of human-built structures that change coastal hydrology, including dikes, berms, levees, ditches, and seawalls. Additionally, transportation networks, including roads and railroads, cause widespread incidental tidal restriction in coastal wetlands. In a first set of national-scale analysis, we have estimated that more than 2.5 million acres of tidal wetlands in the conterminous United States are impounded, tidally-restricted, or drained behind infrastructure. In response to climate change and the accelerating rate of sea level rise, deployment of dikes is expected to increase in the coming decades, with potential for substantial additional impact on wetland ecological condition, on their ability to migrate landward in response to sea level rise, and ultimately the persistence of these vital ecosystems.
Coastal wetlands and mangroves that have been damaged or destroyed can lose their carbon sequestration capacity and the large quantities of carbon stored there can be released into the atmosphere. Improved management of salt marshes and mangroves, leading to enhanced conservation and restoration, is therefore a crucial climate change mitigation strategy—providing both enhanced carbon dioxide removal and avoided methane emissions.
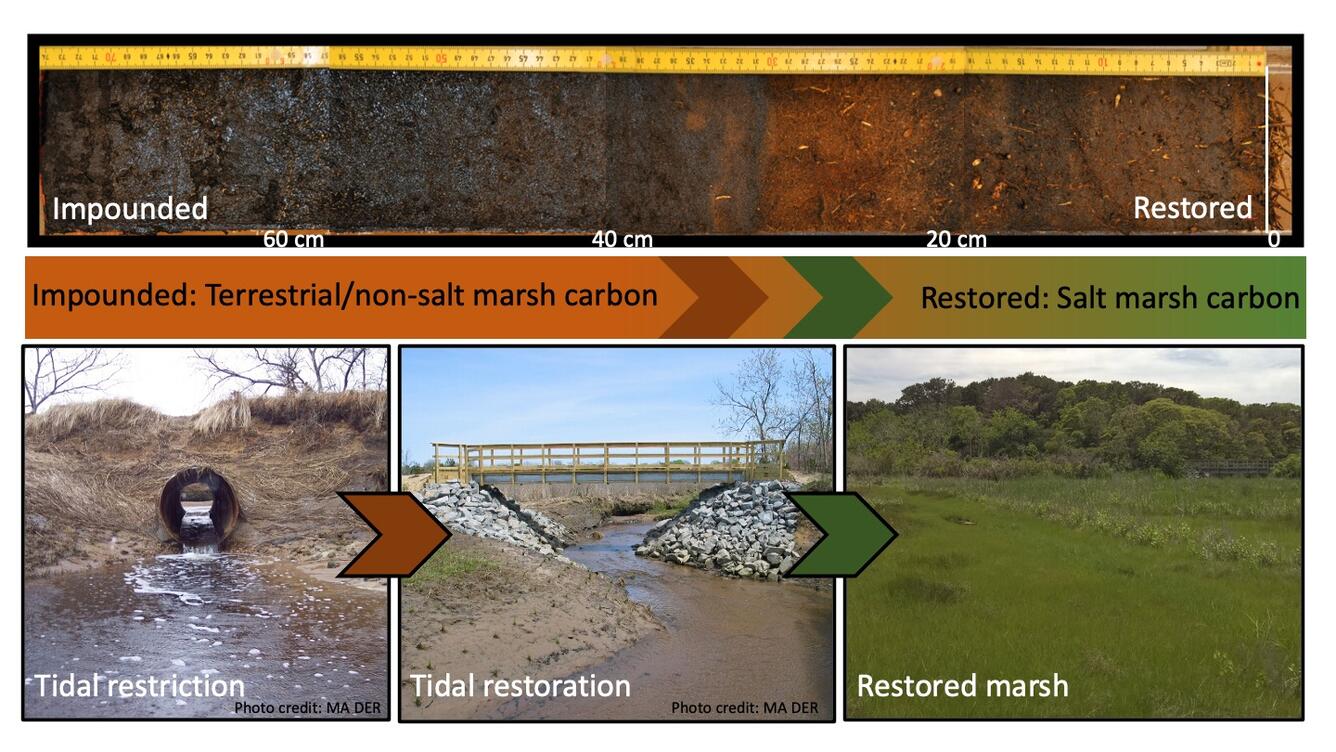
What we do?
The USGS and colleagues will develop functional maps, models and predictions for the existing and future intertidal landscape. Specific tasks include:
- As a part of the NPS Herring River restoration project in the Cape Cod National Seashore, scientists are analyzing the blue carbon value of dike removal and wetland restoration. They are creating site scale models to predict groundwater and surface water hydrological changes in response to sea level rise, biogeochemical and vegetation responses that drive soil elevation change, changes in soil and vegetation carbon stocks, methane emissions and lateral export of carbon to the coastal ocean.
Read more about the project!
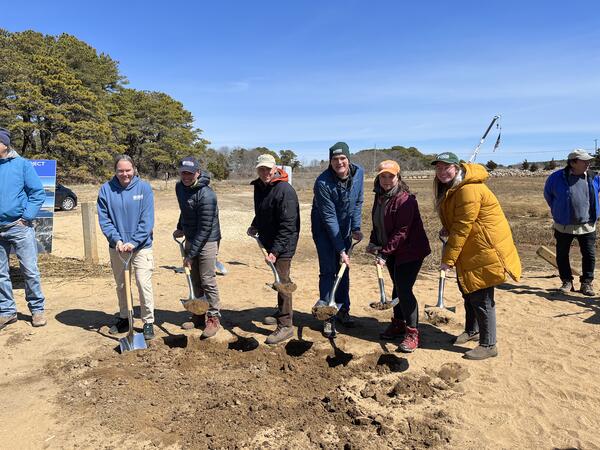
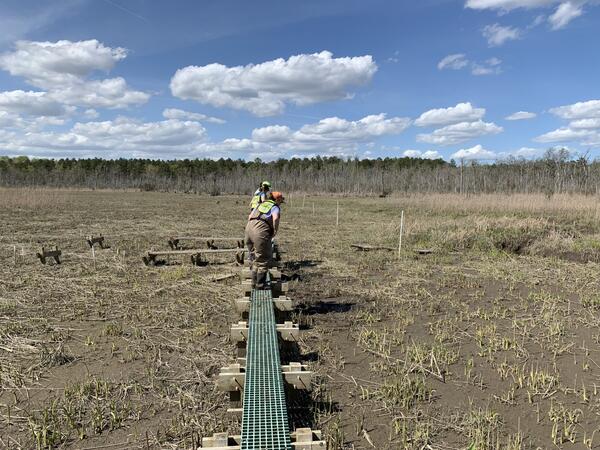
- Mapping wetland management conditions, the suite of structures that interact with coastal wetlands and hydrology. Management condition has not been sufficiently mapped in existing land cover databases which has limited utility to guide blue carbon implementation. These new products will identify degraded wetlands, and opportunities for wetland restoration.
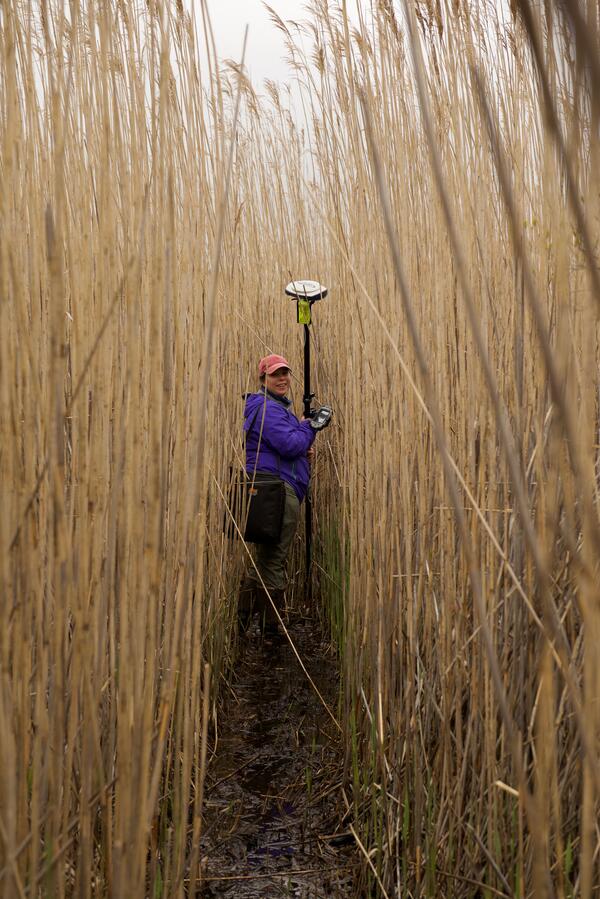
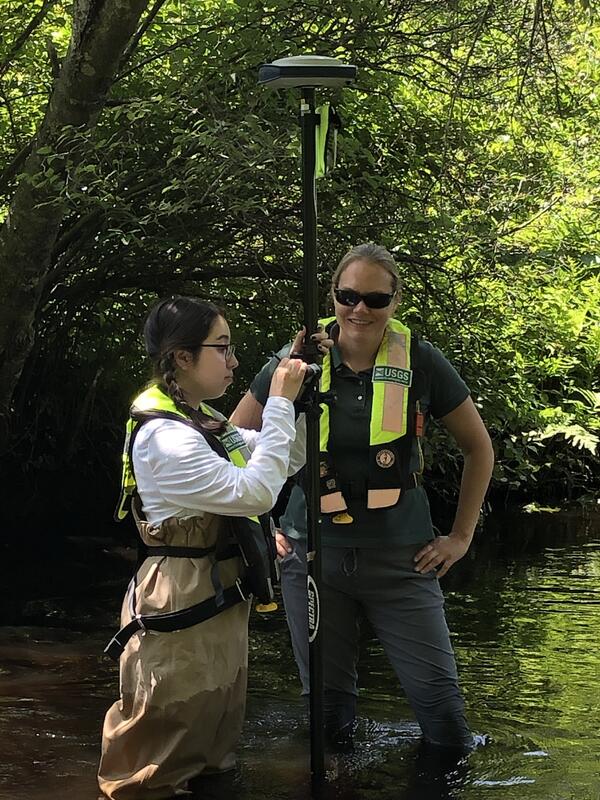
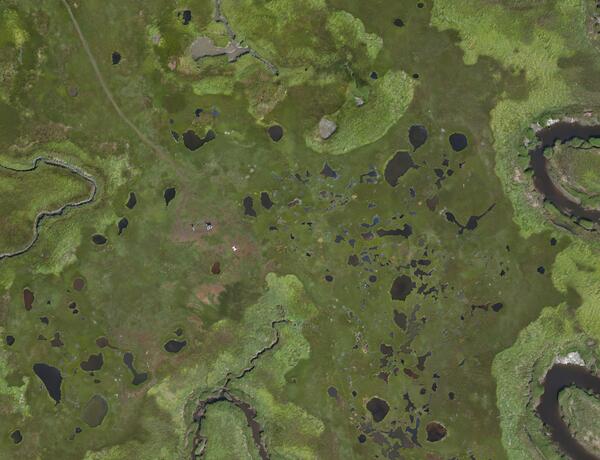
- Use time-series analysis of the full record of Landsat data (beginning in 1986) to detect hydrological condition and tidal connectivity in coastal wetlands, as well as monitor past and future changes in wetland distribution and hydrology.
- Develop national scale models to estimate the amount of carbon exported and alkalinity from tidal wetlands to the coastal ocean, and examine the potential for long-term, millennial-scale, storage in ocean water. Long term storage in seawater is an unrecognized pathway for carbon sequestration/carbon dioxide removal from the atmosphere (CDR). Model implementation will be supported by maps and satellite data to indicate the management condition of tidal wetlands, from local to national scales.
Read more about the project!
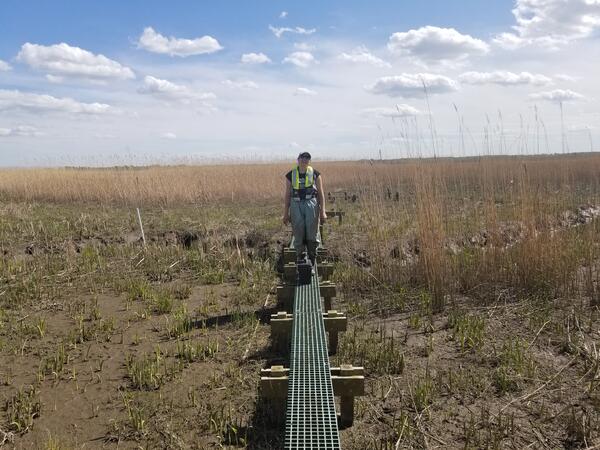
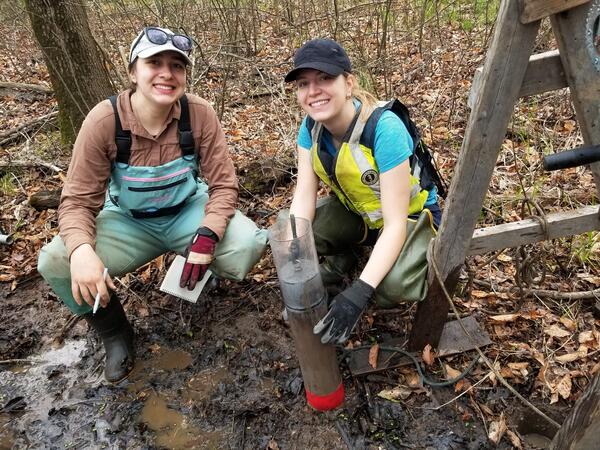
- Examine the potential for enhanced weathering of alkaline minerals in tidal wetlands. These alkaline minerals may be used as an approach to accelerate the export of dissolved carbon and alkalinity, with co-benefits including mitigation of ocean acidification, and providing an elevation subsidy for wetland soils.
Read more about the project!
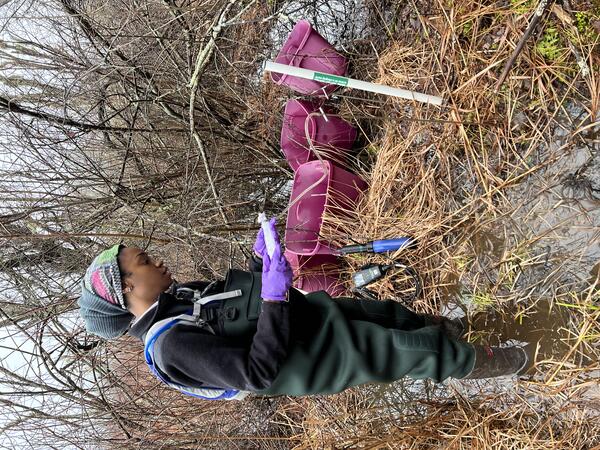
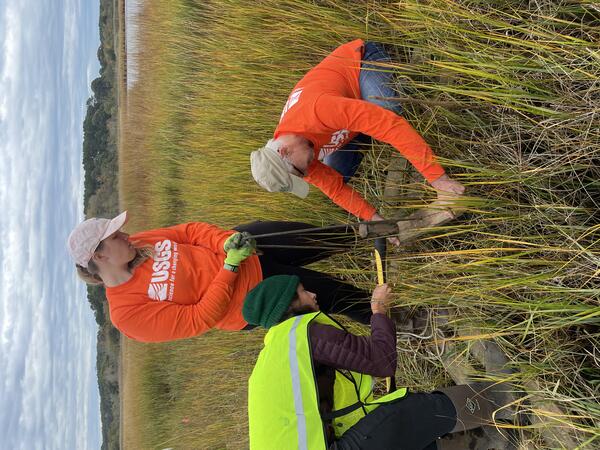
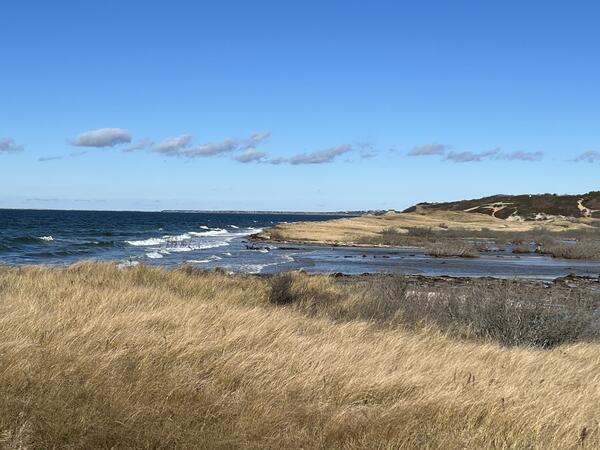
- Evaluate the biogeochemical changes that occur upon reintroduction of salt water to wetlands when historical tidal restriction has resulted in subsidence, acidified soils, and carbon loss. There is limited real world data to inform water quality consequences when there is rewetting of salt marsh peat with seawater. This water quality data can calibrate reactive transport models which inform goals and strategies for adaptive management plans such as water quality and impacts on shellfish beds.
Learn more about the project!
- Quantify environmental and geomorphic drivers of lateral carbon export to the ocean to inform numerical model development as well as provide data for coastal carbon budgets. Although not well known, tidal wetlands remove large amounts of carbon dioxide from the atmosphere, and export the carbon to the coastal ocean. Developing the first predictive model of carbon exports from marshes will provide a tool for predicting future changes in coastal carbon budgets with continued sea level rise.
More on the project!
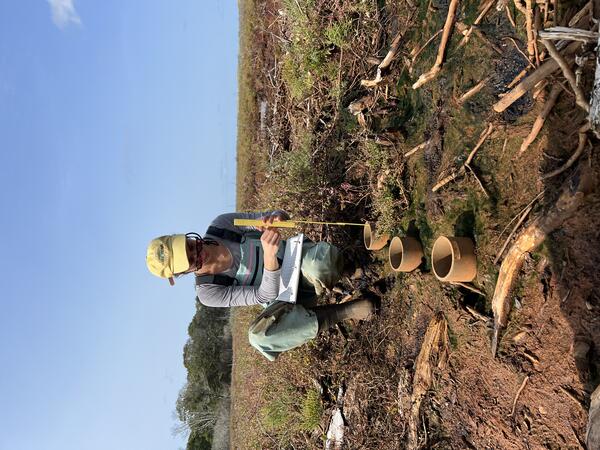
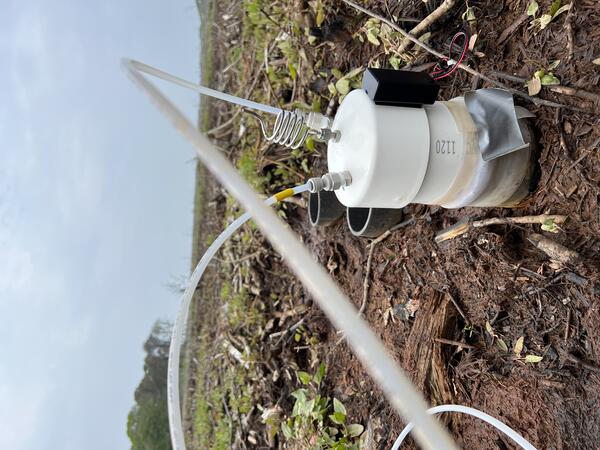
- Assess the net ecosystem carbon budget of wetlands in Louisiana. Wetlands are undergoing a variety of restoration and management actions with the goal to provide tools for managers to plan for future resiliency. As a part of the Deepwater Horizon oil spill restoration effort, the carbon capture of flora, fauna and soils associated with coastal wetland restoration will be evaluated to determine what methods create more resistant marshes with high rates of carbon sequestration.
More on the Gulf Spill Restoration Project.
- Explore drivers of coastal wetland accretion in the face of rising seas and human impacts to understand how burial of organic matter, sediment, and geomorphic evolution of salt marshes is tied to ongoing resilience and associated climate benefits.
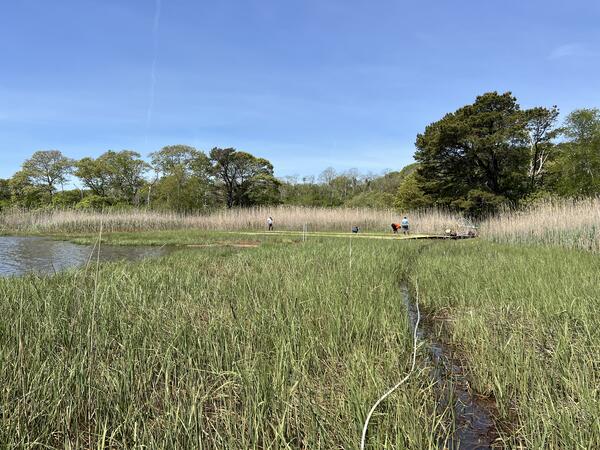
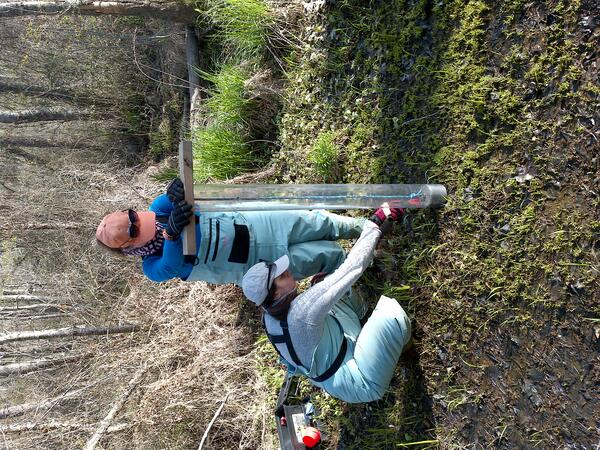
This work is being conducted for current, future, and potential intertidal space throughout the conterminous United States (CONUS) and will include site-, state-, and regional-level analyses.
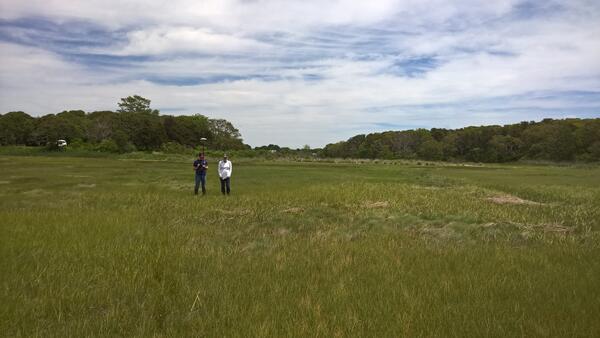
Partners, stakeholders, and end users for this project include the U.S. Fish and Wildlife Service, the National Park Service, state governments and private land managers, and non-governmental organizations. The USGS will continue to actively engage with them to ensure project objectives help meet their specific needs CONUS-wide.
Environmental Geochemistry
The soil core (top) was collected from Bass Creek, Yarmouth, MA, which was restored in 2008. From this soil core, scientists recreated the elevation of the marsh surface over the past 100 years, as well as how quickly elevation changed.
The soil core (top) was collected from Bass Creek, Yarmouth, MA, which was restored in 2008. From this soil core, scientists recreated the elevation of the marsh surface over the past 100 years, as well as how quickly elevation changed.
Carbon dioxide removal experiment led by Kevin Kroeger in Herring River in Wellfleet, Massachusetts.
Carbon dioxide removal experiment led by Kevin Kroeger in Herring River in Wellfleet, Massachusetts.
In collaboration with the National Park Service, Woods Hole Oceanographic Institution, and the Vesta Corporation, the Environmental Geosciences group researched a new approach to climate change mitigation. The team led an experiment at the Herring River site as a first examination.
In collaboration with the National Park Service, Woods Hole Oceanographic Institution, and the Vesta Corporation, the Environmental Geosciences group researched a new approach to climate change mitigation. The team led an experiment at the Herring River site as a first examination.
In collaboration with the National Park Service, Woods Hole Oceanographic Institution, and the Vesta Corporation, the Environmental Geosciences group researched a new approach to climate change mitigation. The team led an experiment at the Herring River site as a first examination.
In collaboration with the National Park Service, Woods Hole Oceanographic Institution, and the Vesta Corporation, the Environmental Geosciences group researched a new approach to climate change mitigation. The team led an experiment at the Herring River site as a first examination.
In collaboration with the National Park Service, Woods Hole Oceanographic Institution, and the Vesta Corporation, the Environmental Geosciences group researched a new approach to climate change mitigation. The team led an experiment at the Herring River site as a first examination.
In collaboration with the National Park Service, Woods Hole Oceanographic Institution, and the Vesta Corporation, the Environmental Geosciences group researched a new approach to climate change mitigation. The team led an experiment at the Herring River site as a first examination.
Image of a tidally restricted wetland in the Herring River Estuary, Cape Cod, MA
Image of a tidally restricted wetland in the Herring River Estuary, Cape Cod, MA
USGS samples water within wetlands to understand which environmental drivers impact carbon cycle and sediment accretion. Here USGS staff (Jennifer O'Keefe Suttles) pumps water from at Phragmites wetland, Mashpee, MA, to analyze at the Woods Hole Coastal and Marine Science Center Environmental Geochemistry lab.
USGS samples water within wetlands to understand which environmental drivers impact carbon cycle and sediment accretion. Here USGS staff (Jennifer O'Keefe Suttles) pumps water from at Phragmites wetland, Mashpee, MA, to analyze at the Woods Hole Coastal and Marine Science Center Environmental Geochemistry lab.
Wetlands play a key role in the coastal carbon cycle, removing carbon dioxide from the atmosphere during photosynthesis and releasing carbon dioxide and methane during respiration. The amount of methane emission is critical to determining how a wetland interacts with the global climate system.
Wetlands play a key role in the coastal carbon cycle, removing carbon dioxide from the atmosphere during photosynthesis and releasing carbon dioxide and methane during respiration. The amount of methane emission is critical to determining how a wetland interacts with the global climate system.
Instruments continue to collect vital data during workplace closures. Here staff perform maintenance on an eddy flux tower located within a phragmites wetland at the Cape Cod National Seashore. The instruments on this tower measure methane and CO2 fluxes related to plant and soil processes day in and day out for the entire year.
Instruments continue to collect vital data during workplace closures. Here staff perform maintenance on an eddy flux tower located within a phragmites wetland at the Cape Cod National Seashore. The instruments on this tower measure methane and CO2 fluxes related to plant and soil processes day in and day out for the entire year.
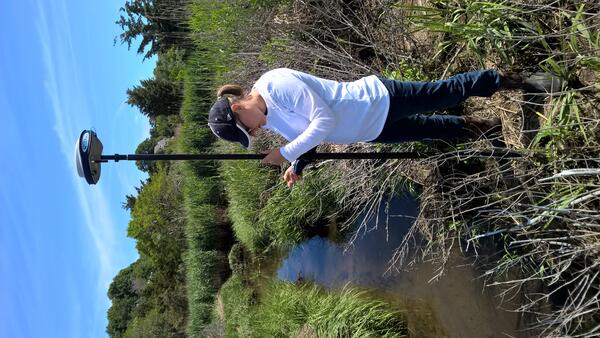
Meagan Eagle, USGS Research Scientist, collecting elevation points in Quivett Creek, Brewster, MA
linkMeagan Eagle, Research Scientists at the U.S. Geological Survey, collects an elevation point along the edge of Quivett Creek in Brewster, MA. This salt marsh was restored in 2005 by replacing a narrow culvert to allow full tidal flow once again.
Meagan Eagle, USGS Research Scientist, collecting elevation points in Quivett Creek, Brewster, MA
linkMeagan Eagle, Research Scientists at the U.S. Geological Survey, collects an elevation point along the edge of Quivett Creek in Brewster, MA. This salt marsh was restored in 2005 by replacing a narrow culvert to allow full tidal flow once again.
Two USGS scientists measure elevation at Bass Creek salt marsh, Yarmouth, MA.
Two USGS scientists measure elevation at Bass Creek salt marsh, Yarmouth, MA.
Salt marsh grass grows in the restored marsh at Bass Creek, Yarmouth, MA.
Salt marsh grass grows in the restored marsh at Bass Creek, Yarmouth, MA.