The 2023 National Seismic Hazard Model – What's Shaking?
No one can predict earthquakes. But existing faults and past earthquakes give us information about future earthquakes, and geology tells us how the ground shakes during an earthquake.
Faults, earthquakes and geology – are the ingredients that go into making a seismic hazard model. Periodically since 1976 the U.S. Geological Survey (USGS) uses the most current data and state-of-the-art forecasting methods to create an updated National Seismic Hazard Model (NSHM). Additionally, engineering analyses inform us about how built structures could react to the ground shaking forecasted by the model. The model and related maps have a surprising variety of uses, from guiding engineering design of structures to helping determine earthquake insurance rates, and informing government officials, emergency managers, and public officials about potential risks to their communities. The distinction between hazard and risk is that hazard refers to natural phenomena from earthquakes (e.g., ground shaking), whereas risk refers to consequences of the hazard (e.g., dangers the hazard poses to life and property). The USGS is currently working on the updated hazard model for the 50 states targeted for completion and release in 2023. Let’s look at what goes into the NSHM in more detail to better appreciate the improvements and progress for the 2023 model.
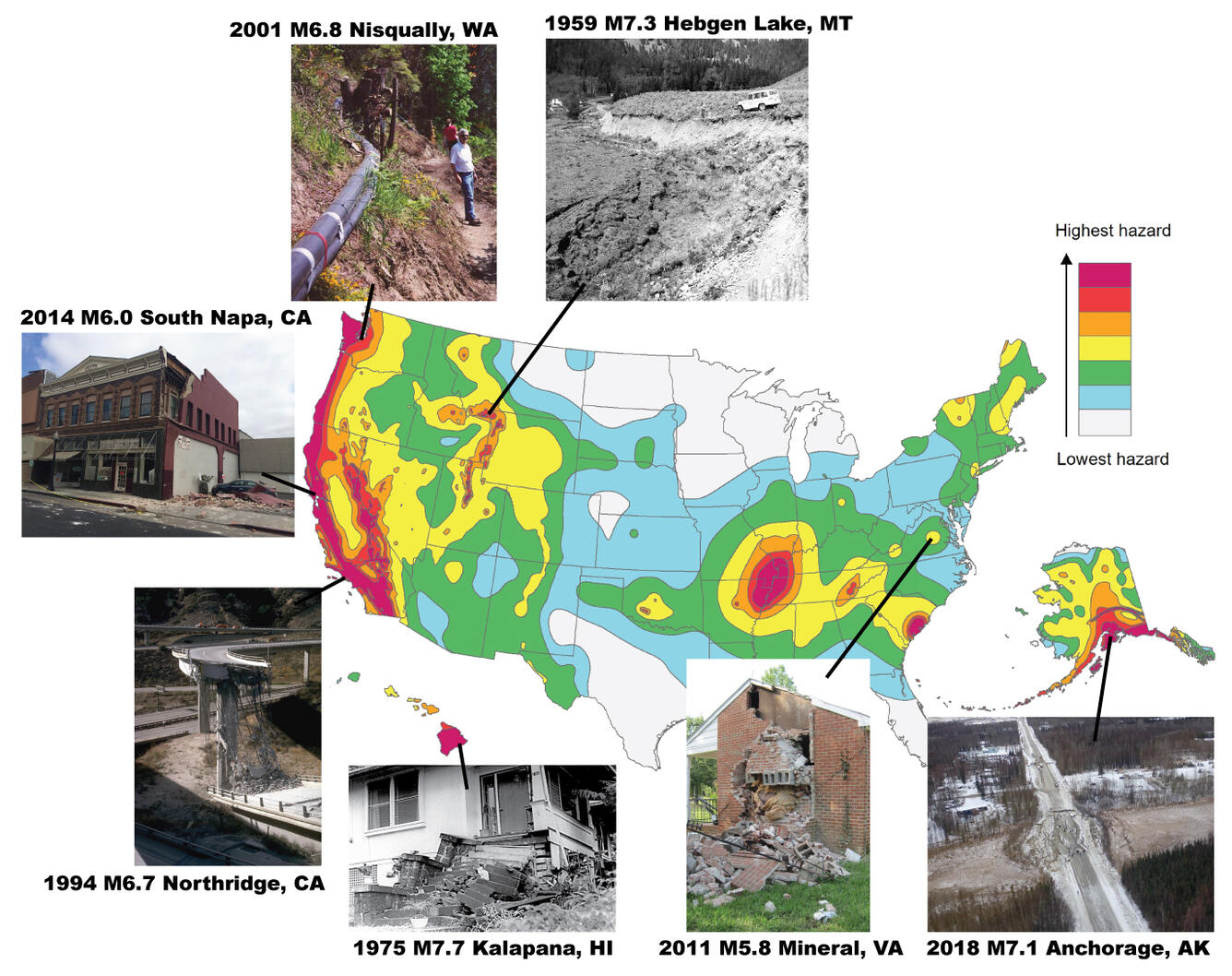
Faults and Earthquakes
Existing faults and locations of past earthquakes are the starting point for a seismic hazard model. The location of faults is known through observations of topographic and landscape disruptions caused by movement on said faults. Earthquakes on these faults disrupt the surface features, and the date the earthquake occurred can be estimated by the age of the disrupted features. For example, if we know that a certain sediment deposit is 1,000 years old, and we see that this sediment has been cut and moved by the fault, we know that an earthquake on the fault reached the surface in the last 1,000 years. These observations can be made in the field or using remotely sensed imagery, like aerial imagery and other techniques. Unfortunately, earthquakes don’t just happen on mapped faults – they occur all the time in places where there is no fault on the map – but all those earthquakes are also taken into account in hazard models, as indicators of where and how often earthquakes could occur away from known faults.
In addition to the fault locations, we need to know how active each fault is, which reflects how frequently a fault has earthquakes and how big those earthquakes may be. These activity rates can be measured both by geologists, with knowledge of how much displacement has occurred along a fault over a given length of time (like our previous example of a displaced sediment layer with a known age), and by geodesists. For example, geodesists use high-precision Global Navigation Satellite System (GNSS) instruments distributed across the western United States to determine the velocities of the Earth’s surface relative to the stable and very slowly deforming eastern United States. Although earthquakes are rare in the central and eastern United States, they do occur and can cause damage (for example, the 2011 M5.8 earthquake in Mineral, Virginia).
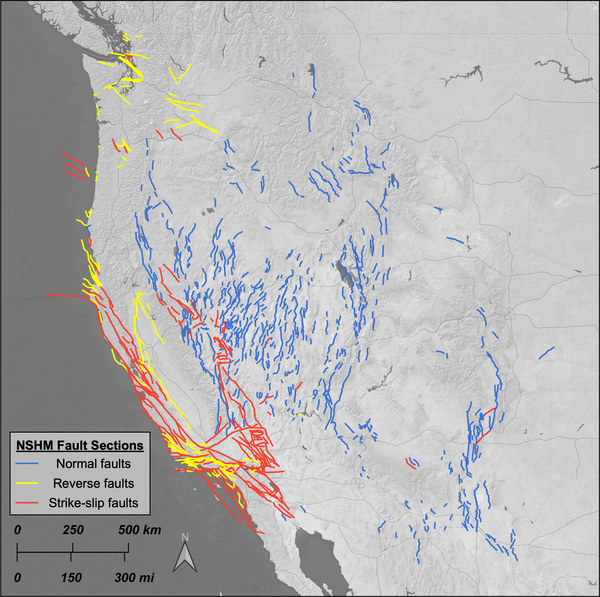
Combining fault locations and activity rates allows us to calculate the frequency of rupture along a given fault. The yearly frequencies of rupture are very low, so this is translated into a percent chance of a fault rupturing over a longer given time scale (for example, 10% in 50 years). This process of calculating times between earthquakes (known as recurrence) also considers the possibility of earthquakes jumping from one fault to another, as has been observed in past earthquakes such as the 1992 M7.3 Landers earthquake in the Mojave Desert of southern California. While the chances of earthquakes on a given fault are very small over short time scales like years to decades, it is important to remember that the chance of an earthquake is generally higher in California, especially near the San Andreas fault, than in areas with fewer active faults, such as Colorado.
Ground Motions
Once we’ve gathered all the best data for fault locations and how often they generate earthquakes, we want to know the level of shaking that each earthquake is likely to produce.
The ground shaking caused by fault rupture can be measured worldwide by very sensitive instruments called seismometers, but it is nearest the fault where shaking has the greatest human impact. The shaking intensity at a particular location is primarily related to: 1) the magnitude (or size) of the earthquake, 2) the distance from the fault, 3) the type of soil or rock present, and 4) the depth of bedrock. All these factors combine and trade off to generate combinations of very sudden jolts of shaking (high-frequency waves) and much slower, rocking or rolling waves (low-frequency waves). High-frequency waves can rattle short buildings (e.g., 1 to 2 stories), dams, and nuclear power plants, crack stiff walls, and topple or slide building contents that are not anchored to the structure, such as appliances or furniture. Low-frequency waves can especially affect tall buildings or long bridges, causing them to sway. Localized areas of structural damage can be caused by soft and deep soils or sudden changes in geology that amplify the shaking intensity.
Databases of past ground motions are used to make models of future potential ground shaking. When combined with fault locations and earthquake frequencies, a hazard model forecasts the chances of low- and high-frequency ground shaking intensities at any location of interest. As described below, these hazards are fed into engineering project needs and construction code applications to help improve designs of new structures or guide the retrofitting plans of old ones.
Engineering & Risk
Engineering and risk research extends the hazard model to practical civil engineering applications and provides feedback for improving the hazard model. Traditionally, the NSHM serves as a starting point for designing earthquake-resistant structures. For buildings, structural engineers need to design beams, columns, and connections of buildings to withstand shaking during an earthquake. The building codes used for these designs are based on forecasted ground motion intensities derived from the NSHM. Besides collaborating with building code partners for designing buildings, such as the Building Seismic Safety Council (BSSC), USGS engineers and scientists also collaborate on designs for other structures, such as bridges with the American Association of State Highway and Transportation Officials (AASHTO) and railways with the American Railway Engineering and Maintenance-of-Way Association (AREMA).
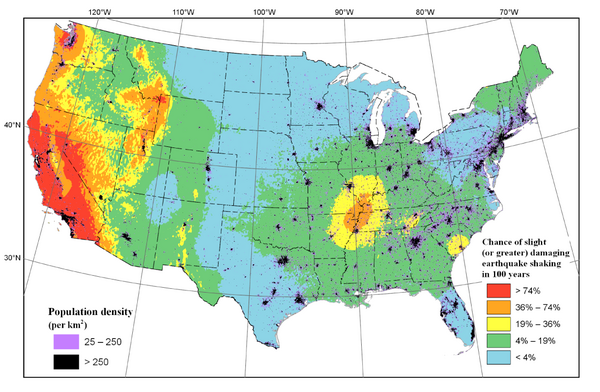
In addition to engineering design, the USGS engineers and scientists also collaborate with partners on extending the hazard forecasts of ground shaking to risk forecasts of consequent damage to buildings and other structures. For example, USGS and the Federal Emergency Management Agency (FEMA) have forecasted seismic damage to the nation’s buildings in terms of yearly repair costs. Furthermore, the insurance industry uses the NSHM to assess seismic risk and determine insurance premiums.
What’s new for 2023?
As more observations are made, our knowledge of active faults increases. To capture all of what we know about active faults across the U.S., we are reviewing new reports and literature published since the previous update to the NSHM fault model in 2014. Additionally, given improvements made between 2014 and now in computational power, modeling algorithms, and the availability of more data, we can incorporate more faults than in previous models – over 1,000 for the 2023 NSHM, whereas the previous model used about 640. This reduces our uncertainty on where earthquakes may happen in the future, and thereby increases the precision of the resulting seismic hazard model.
The 2023 NSHM will include recently updated ground motion models for subduction-zone faults (present in the Pacific Northwest and Alaska) as part of its updated national hazard assessment. The Hawaii portion of the NSHM has already been updated to reflect, in part, a swath of new earthquakes related to volcanic eruptions that have changed the way we understand ground shaking on the Island of Hawai‘i. The 2023 update for the Lower 48 states will also focus on better characterizing shaking within sedimentary basins, large geological depressions generally filled with soft sediments down to deep bedrock, where major urban areas are often centered. New studies in southern and central California, Seattle, Portland, Reno, and Las Vegas will be performed to determine if these basin regions need special treatment within the NSHM.
To broaden the utility of the NSHM beyond traditional design and assessment of buildings, USGS engineers and scientists are currently exploring potential applications that assess seismic risk for other critical infrastructure systems such as gas pipelines, highway bridges, and dams. Other engineering and risk research efforts related to the NSHM include: 1) developing earthquake scenarios for response planning, 2) extending the ground shaking forecast to ground failures such as soil liquefaction that can cause additional damage, and 3) studying the interactions of multiple hazards for engineering and risk assessments of infrastructure systems.
While incomplete, the preceding examples aim to illustrate the many different improvements to an already useful forecast of future earthquake shaking and its consequences in the United States.
~ written by Alex Hatem, Sean Ahdi, N. Simon Kwong, and Lisa Wald, June 15, 2022
For More Information
- Earthquake Hazards Program - Hazards
- Earthquake geology inputs for National Seismic Hazard Model (NSHM) 2023
Acknowledgements
The authors thank Allison Shumway, Rob Chase, and Andrew Makdisi for their suggestions for photographs and help with the first image. We also thank Nico Luco and Leah Salditch for their reviews that greatly improved the readability.
The Scientists Behind the Science
Note: Many scientists are part of this effort. The information below is only for those who wrote this article.
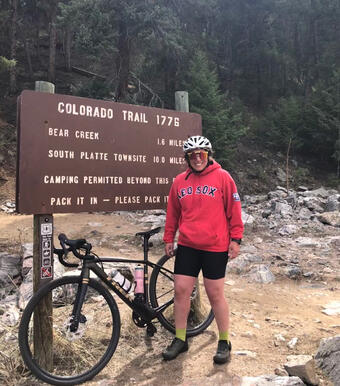
Alex Hatem is a research geologist and has been with the USGS Geologic Hazards Science Center since 2019. Alex is interested in active faulting, fault evolution through space and time, and the recent history of earthquakes over the past ~20,000 years. Alex works on field projects to understand the parameters of active faults up-close-and-personal, and then uses that data to inform systems-level efforts like the National Seismic Hazard Model. When not thinking about active faulting, Alex likes to take in the local geomorphology via biking, skiing, or walking her dogs.
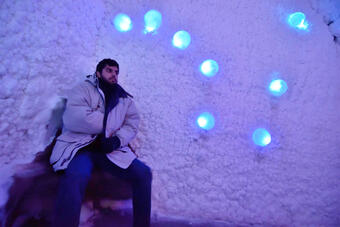
Sean Ahdi is a research geophysicist at the USGS Geologic Hazards Science Center. Leveraging an interdisciplinary background integrating geology, seismology, and geophysics into civil, geotechnical, and earthquake engineering applications, Sean’s research is aimed at improving our understanding of how different types of rocks and soils affect ground shaking levels that humans and built infrastructure may experience during earthquakes. In his free time, Sean enjoys exploring the geology and nature in Colorado across its four seasons, especially with all the snow that he did not get to experience while growing up in southern California.
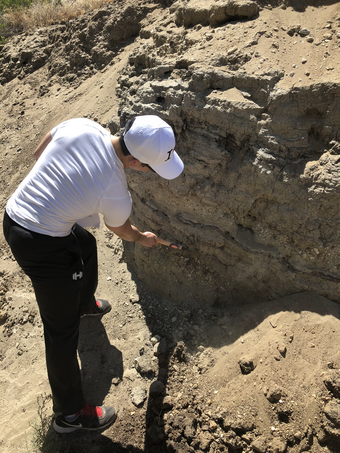
N. Simon Kwong is a research civil engineer at the USGS Geologic Hazards Science Center. His research focuses on extending USGS earthquake science for assessing and mitigating seismic risks to critical infrastructure systems. For this purpose, he helps end users understand the variety of uncertainties that appear in different contexts such as probabilistic seismic hazard analyses, nonlinear dynamic structural analyses, and national seismic risk assessments. Outside of work, Simon likes to explore wellness through experimentation with diverse foods and physical activities.
No one can predict earthquakes. But existing faults and past earthquakes give us information about future earthquakes, and geology tells us how the ground shakes during an earthquake.
Faults, earthquakes and geology – are the ingredients that go into making a seismic hazard model. Periodically since 1976 the U.S. Geological Survey (USGS) uses the most current data and state-of-the-art forecasting methods to create an updated National Seismic Hazard Model (NSHM). Additionally, engineering analyses inform us about how built structures could react to the ground shaking forecasted by the model. The model and related maps have a surprising variety of uses, from guiding engineering design of structures to helping determine earthquake insurance rates, and informing government officials, emergency managers, and public officials about potential risks to their communities. The distinction between hazard and risk is that hazard refers to natural phenomena from earthquakes (e.g., ground shaking), whereas risk refers to consequences of the hazard (e.g., dangers the hazard poses to life and property). The USGS is currently working on the updated hazard model for the 50 states targeted for completion and release in 2023. Let’s look at what goes into the NSHM in more detail to better appreciate the improvements and progress for the 2023 model.
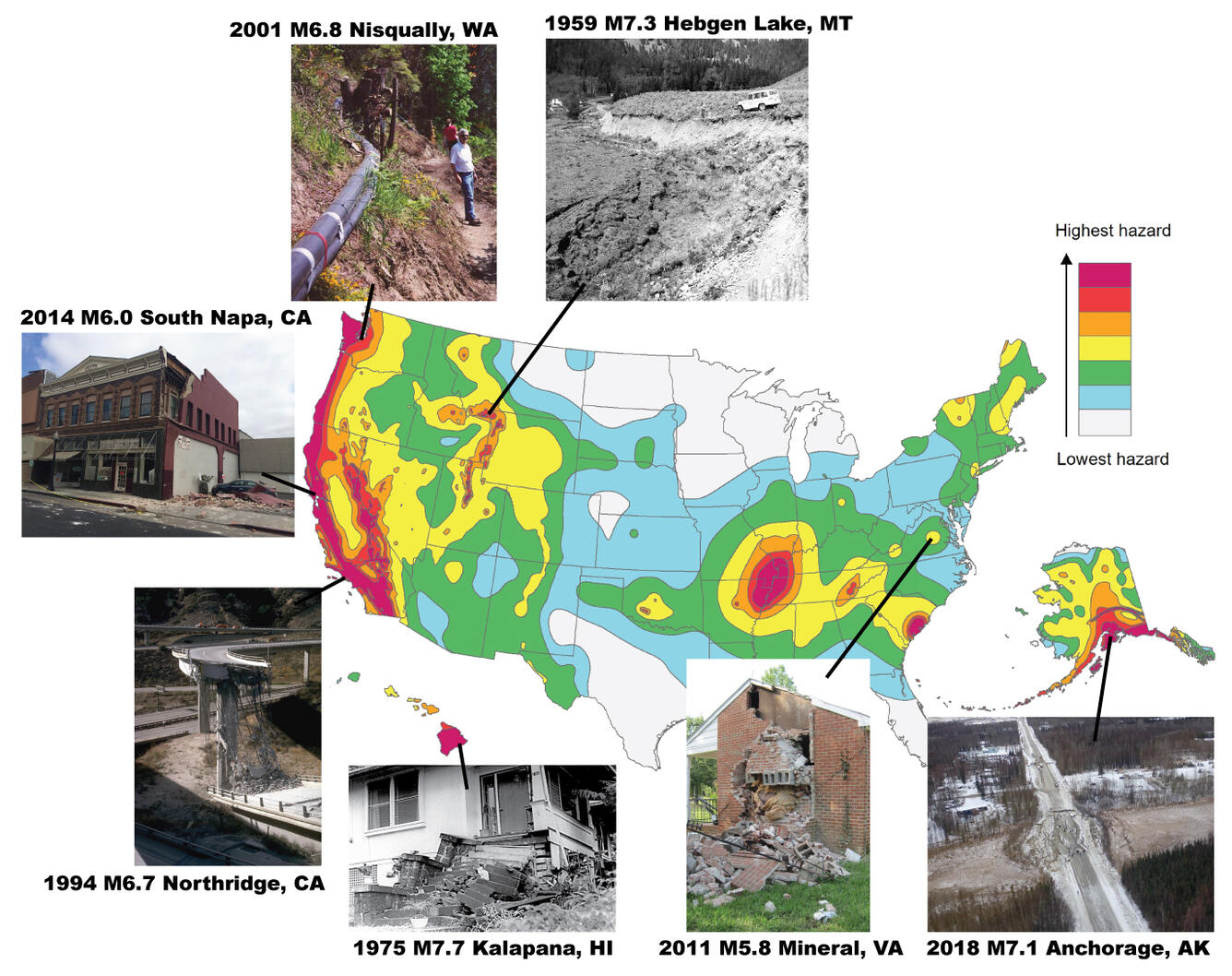
Faults and Earthquakes
Existing faults and locations of past earthquakes are the starting point for a seismic hazard model. The location of faults is known through observations of topographic and landscape disruptions caused by movement on said faults. Earthquakes on these faults disrupt the surface features, and the date the earthquake occurred can be estimated by the age of the disrupted features. For example, if we know that a certain sediment deposit is 1,000 years old, and we see that this sediment has been cut and moved by the fault, we know that an earthquake on the fault reached the surface in the last 1,000 years. These observations can be made in the field or using remotely sensed imagery, like aerial imagery and other techniques. Unfortunately, earthquakes don’t just happen on mapped faults – they occur all the time in places where there is no fault on the map – but all those earthquakes are also taken into account in hazard models, as indicators of where and how often earthquakes could occur away from known faults.
In addition to the fault locations, we need to know how active each fault is, which reflects how frequently a fault has earthquakes and how big those earthquakes may be. These activity rates can be measured both by geologists, with knowledge of how much displacement has occurred along a fault over a given length of time (like our previous example of a displaced sediment layer with a known age), and by geodesists. For example, geodesists use high-precision Global Navigation Satellite System (GNSS) instruments distributed across the western United States to determine the velocities of the Earth’s surface relative to the stable and very slowly deforming eastern United States. Although earthquakes are rare in the central and eastern United States, they do occur and can cause damage (for example, the 2011 M5.8 earthquake in Mineral, Virginia).
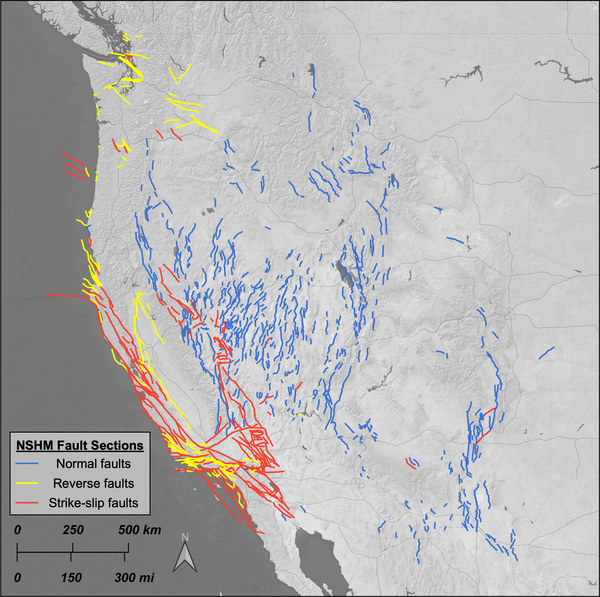
Combining fault locations and activity rates allows us to calculate the frequency of rupture along a given fault. The yearly frequencies of rupture are very low, so this is translated into a percent chance of a fault rupturing over a longer given time scale (for example, 10% in 50 years). This process of calculating times between earthquakes (known as recurrence) also considers the possibility of earthquakes jumping from one fault to another, as has been observed in past earthquakes such as the 1992 M7.3 Landers earthquake in the Mojave Desert of southern California. While the chances of earthquakes on a given fault are very small over short time scales like years to decades, it is important to remember that the chance of an earthquake is generally higher in California, especially near the San Andreas fault, than in areas with fewer active faults, such as Colorado.
Ground Motions
Once we’ve gathered all the best data for fault locations and how often they generate earthquakes, we want to know the level of shaking that each earthquake is likely to produce.
The ground shaking caused by fault rupture can be measured worldwide by very sensitive instruments called seismometers, but it is nearest the fault where shaking has the greatest human impact. The shaking intensity at a particular location is primarily related to: 1) the magnitude (or size) of the earthquake, 2) the distance from the fault, 3) the type of soil or rock present, and 4) the depth of bedrock. All these factors combine and trade off to generate combinations of very sudden jolts of shaking (high-frequency waves) and much slower, rocking or rolling waves (low-frequency waves). High-frequency waves can rattle short buildings (e.g., 1 to 2 stories), dams, and nuclear power plants, crack stiff walls, and topple or slide building contents that are not anchored to the structure, such as appliances or furniture. Low-frequency waves can especially affect tall buildings or long bridges, causing them to sway. Localized areas of structural damage can be caused by soft and deep soils or sudden changes in geology that amplify the shaking intensity.
Databases of past ground motions are used to make models of future potential ground shaking. When combined with fault locations and earthquake frequencies, a hazard model forecasts the chances of low- and high-frequency ground shaking intensities at any location of interest. As described below, these hazards are fed into engineering project needs and construction code applications to help improve designs of new structures or guide the retrofitting plans of old ones.
Engineering & Risk
Engineering and risk research extends the hazard model to practical civil engineering applications and provides feedback for improving the hazard model. Traditionally, the NSHM serves as a starting point for designing earthquake-resistant structures. For buildings, structural engineers need to design beams, columns, and connections of buildings to withstand shaking during an earthquake. The building codes used for these designs are based on forecasted ground motion intensities derived from the NSHM. Besides collaborating with building code partners for designing buildings, such as the Building Seismic Safety Council (BSSC), USGS engineers and scientists also collaborate on designs for other structures, such as bridges with the American Association of State Highway and Transportation Officials (AASHTO) and railways with the American Railway Engineering and Maintenance-of-Way Association (AREMA).
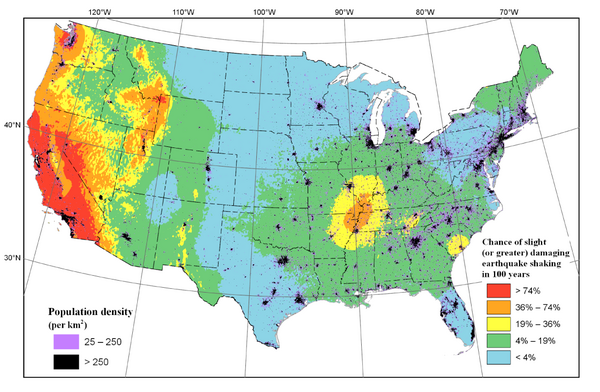
In addition to engineering design, the USGS engineers and scientists also collaborate with partners on extending the hazard forecasts of ground shaking to risk forecasts of consequent damage to buildings and other structures. For example, USGS and the Federal Emergency Management Agency (FEMA) have forecasted seismic damage to the nation’s buildings in terms of yearly repair costs. Furthermore, the insurance industry uses the NSHM to assess seismic risk and determine insurance premiums.
What’s new for 2023?
As more observations are made, our knowledge of active faults increases. To capture all of what we know about active faults across the U.S., we are reviewing new reports and literature published since the previous update to the NSHM fault model in 2014. Additionally, given improvements made between 2014 and now in computational power, modeling algorithms, and the availability of more data, we can incorporate more faults than in previous models – over 1,000 for the 2023 NSHM, whereas the previous model used about 640. This reduces our uncertainty on where earthquakes may happen in the future, and thereby increases the precision of the resulting seismic hazard model.
The 2023 NSHM will include recently updated ground motion models for subduction-zone faults (present in the Pacific Northwest and Alaska) as part of its updated national hazard assessment. The Hawaii portion of the NSHM has already been updated to reflect, in part, a swath of new earthquakes related to volcanic eruptions that have changed the way we understand ground shaking on the Island of Hawai‘i. The 2023 update for the Lower 48 states will also focus on better characterizing shaking within sedimentary basins, large geological depressions generally filled with soft sediments down to deep bedrock, where major urban areas are often centered. New studies in southern and central California, Seattle, Portland, Reno, and Las Vegas will be performed to determine if these basin regions need special treatment within the NSHM.
To broaden the utility of the NSHM beyond traditional design and assessment of buildings, USGS engineers and scientists are currently exploring potential applications that assess seismic risk for other critical infrastructure systems such as gas pipelines, highway bridges, and dams. Other engineering and risk research efforts related to the NSHM include: 1) developing earthquake scenarios for response planning, 2) extending the ground shaking forecast to ground failures such as soil liquefaction that can cause additional damage, and 3) studying the interactions of multiple hazards for engineering and risk assessments of infrastructure systems.
While incomplete, the preceding examples aim to illustrate the many different improvements to an already useful forecast of future earthquake shaking and its consequences in the United States.
~ written by Alex Hatem, Sean Ahdi, N. Simon Kwong, and Lisa Wald, June 15, 2022
For More Information
- Earthquake Hazards Program - Hazards
- Earthquake geology inputs for National Seismic Hazard Model (NSHM) 2023
Acknowledgements
The authors thank Allison Shumway, Rob Chase, and Andrew Makdisi for their suggestions for photographs and help with the first image. We also thank Nico Luco and Leah Salditch for their reviews that greatly improved the readability.
The Scientists Behind the Science
Note: Many scientists are part of this effort. The information below is only for those who wrote this article.
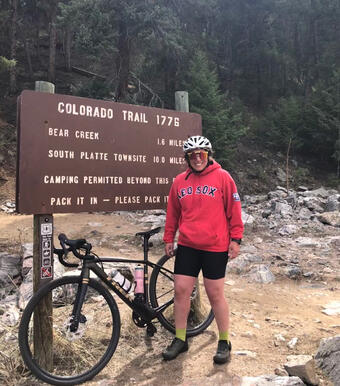
Alex Hatem is a research geologist and has been with the USGS Geologic Hazards Science Center since 2019. Alex is interested in active faulting, fault evolution through space and time, and the recent history of earthquakes over the past ~20,000 years. Alex works on field projects to understand the parameters of active faults up-close-and-personal, and then uses that data to inform systems-level efforts like the National Seismic Hazard Model. When not thinking about active faulting, Alex likes to take in the local geomorphology via biking, skiing, or walking her dogs.
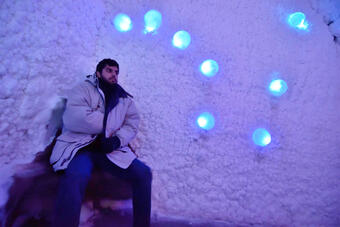
Sean Ahdi is a research geophysicist at the USGS Geologic Hazards Science Center. Leveraging an interdisciplinary background integrating geology, seismology, and geophysics into civil, geotechnical, and earthquake engineering applications, Sean’s research is aimed at improving our understanding of how different types of rocks and soils affect ground shaking levels that humans and built infrastructure may experience during earthquakes. In his free time, Sean enjoys exploring the geology and nature in Colorado across its four seasons, especially with all the snow that he did not get to experience while growing up in southern California.
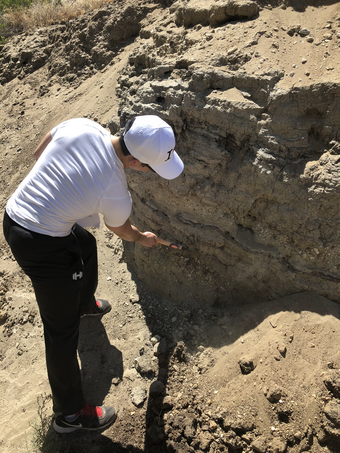
N. Simon Kwong is a research civil engineer at the USGS Geologic Hazards Science Center. His research focuses on extending USGS earthquake science for assessing and mitigating seismic risks to critical infrastructure systems. For this purpose, he helps end users understand the variety of uncertainties that appear in different contexts such as probabilistic seismic hazard analyses, nonlinear dynamic structural analyses, and national seismic risk assessments. Outside of work, Simon likes to explore wellness through experimentation with diverse foods and physical activities.