Rocks and Paleomagnetics Laboratory
Building 16, CalVO contact Duane Champion
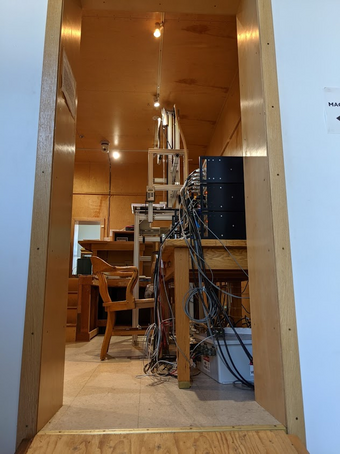
Researchers at the USGS Paleomagnetics Laboratory study the magnetic record contained in rocks and other natural materials. Their research ranges from the small – signal-carrying minerals within specimens – to the large – regional aeromagnetic surveys and continent-scale tectonic movements. The past variations of the geomagnetic field make paleomagnetism a precise, powerful tool which applies to a wide variety of important geological questions.
- Motions of the Earth’s crust. Paleomagnetic data provide the basic framework for plate tectonic theory. They can be used to understand the geological history of regions, such as in assessing earthquake hazards and fault activity in structurally complex regions. Aeromagnetic data reveal structures and rock unit contacts.
- Ages of rocks. Paleomagnetic data can help determine the frequency and duration of volcanic eruptions. They can aid in the mapping of active volcanoes by correlating rocks. The paleomagnetism of mineral deposits can assist in figuring out their age.
- Behavior of the geomagnetic field. Earth’s magnetic field shields us from dangerous solar radiation. Paleomagnetic data help determine the speed and duration of polarity reversals, when the geomagnetic shield becomes small. The strength of the field through time, paleointensity, can be a correlative tool as well as affecting some radioisotopes.
Facilities and Equipment
History of the Laboratory
Paleomagnetism and Magnetic Techniques
Active Personnel:
Duane Champion - dchamp@usgs.gov (650) 329-4671
Jonathan Glen - jglen@usgs.gov (650) 329-5282
Anthony Pivarunas – apivarunas@usgs.gov
Margaret Avery – mavery@usgs.gov
Affiliated and Emeriti:
Jonathan Hagstrum
Rick Blakely
Ray Wells
Ed Mankinen
Jack Hillhouse
Sherman Gromme
Michel Prevot
Norman Watkins
Partners: Caltech, Stanford, UC Santa Cruz, UC Berkeley, Berkeley Geochronology Center, RAPID Consortium, University of Florida, SUNY College at Geneseo
The Paleomagnetics Laboratory is presently located in Building 16 at the Menlo Park campus and contains a wide variety of instruments used to analyze paleomagnetism and rock magnetic properties. The data acquired here support many mission related USGS activities. The most recent addition to the laboratory is a fully automatic cryogenic magnetometer with in-line alternating field demagnetization capability. It is capable of precise and rapid measurement of materials with a wide range of magnetic signal intensity to determine their original magnetizations as well as any superimposed secondary magnetizations.
Specific Equipment
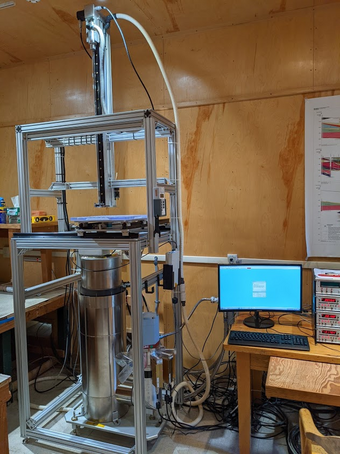
- Superconducting cryogenic magnetometer: This instrument uses three SQUIDs (superconducting quantum interference devices) to measure the magnetizations of specimens and has a wide practical sensitivity from 1 emu to 5 x 10-8 emu. It is capable of automated measurement and alternating-field demagnetization routines as well as a variety of rock magnetic tests. Typical measurement time per sample is under 2 minutes; data are logged and reduced on a computer and are cross-compatible with a variety of analytical software.
- Demagnetization equipment, alternating-field (AF) and thermal: An AF unit is located in-line with the cryogenic magnetometer setup. It can produce a maximum magnetic field of 800 Gauss, that is, over 1000 times the Earth’s magnetic field. This ‘cleans’ secondary magnetization from samples. Thermal demagnetization is carried out in a shielded furnace which can reach 700 °C and holds up to 48 standard specimens per 1-hour heating/cooling cycle conducted. Both demagnetization devices are housed in a shielded room, minimizing magnetic acquisition during measurements.
- MFK1 Kappabridge: This instrument measures magnetic susceptibility at high, low, and room temperatures. This helps determine rock fabrics (from deformation lava flows, or paleo-currents) and identify magnetic minerals which carry the remanent signal in our specimens.
- Shielded room: The magnetometer, demagnetization equipment, and samples undergoing analysis are housed in a magnetically shielded room that reduces the ambient field to 1% of its normal value. This room is wrapped in multiple layers of transformer steel to achieve this effect. The weak field in this room reduces measurement time, and minimizes contamination from stray magnetic fields in both samples and equipment.
- Curie balance Strong-field magnetization and Curie temperatures are measured in this electromagnet/balance system. Tiny specimens (typically 0.5-1.0 gram; solid or granular) are suspended between the electromagnet pole pieces which produce a magnetic gradient force on the specimen. A balance automatically records apparent weight, which is proportional to magnetization, as the specimen is heated in air, vacuum, or an inert gas. The electromagnet field limit is 8000 Gauss (0.8 Tesla). A complete heating/cooling cycle up to 700 degrees C runs in 2.25 hours. This instrument is an important piece of our lab’s history, designed and built in-house by Allan Cox and Richard Doell (see History of the Laboratory). Have you ever used a rice cooker? It turns out that rice cookers also use a similar Curie balance principle.
-
Sources/Usage: Public Domain. View Media Details
This instrument is an electromagnet that is used for measuring the variation of induced magnetization in standard-sized paleomagnetic samples. Applied fields range from 0 to 700 milliTesla. Electromagnet: This instrument can overwhelm a specimen’s original magnetization with a large 7000 Gauss magnetic field at room temperature, known as an Isothermal Remanent Magnetization (IRM). A similar process happens in nature during lightning strikes! This machine helps lets researchers determine saturation remanent magnetization and coercivity of remanence to identify rock magnetic mineralogy.
- Rock drills and other field equipment: The laboratory maintains a full complement of portable rock drills, sample orienting equipment, sediment sampling tools, and double-bladed diamond saws to prepare samples.
Pioneering research on the geomagnetic polarity time scale performed in the original laboratory was instrumental in confirming the hypothesis of seafloor spreading and led to the development of the theory of plate tectonics. During 1959-1966, the scientists of the Rock Magnetics Project in Menlo Park were involved in a race to prove that reverse-polarity magnetization in rocks was the result of global reversals of the geomagnetic field, and not due to variation of individual rock magnetic properties. To prove their case, the scientists had to show that rocks of the same age throughout the world had the same magnetic polarity. They collected samples from Alaska to Antarctica and from Hawaii to New Mexico. In the laboratory, they measured the magnetic polarity of rock samples with a spinner magnetometer and determined the ages of samples with an argon mass spectrometer. As more and more polarity-age pairs were gathered at this laboratory and elsewhere, a consistent pattern began to emerge, demonstrating that indeed the earth’s magnetic field had reversed polarity many times in the past. Within a few years, the Geomagnetic Polarity Reversal Timescale was published. When it was recognized that the polarity timescale was matched by magnetic patterns on the seafloor, the concepts of seafloor-spreading and the mobility of continents became widely accepted. This discovery about earth magnetism became the foundation for modern plate tectonic models that explain mountain building, the formation of ocean basins, and the distribution of continents.
In 1995, recognition of the fundamental scientific contributions made by Allan Cox, Richard Doell, and Brent Dalrymple from 1959 to 1966 led to listing of the Rock Magnetics Laboratory on the National Register of Historic Places (#94001647). The original laboratory was in a neglected storage building previously used as a laundry for Dibble Army Hospital during World War II. Cox and Doell established their Rock Magnetics Laboratory in this “tarpaper shack” in 1959, soon after the USGS acquired the army site. The nearly all-wood construction of the building was ideal for their purposes. Construction of the new laboratory in 1996 required removal of the original building, the essence of which is presently preserved in an exhibit in Building 16. A documentary video, “Secrets in Stone,” was also commissioned as part of the exhibit. The 30-minute video chronicles events from the discovery of geomagnetic reversals to the acceptance of plate tectonics and explains the essential scientific principles in terms understandable to a general audience. Colorful animations and interviews with some of the scientists involved in this scientific discovery bring the story to life for viewers. The movie is available in DVD format; contact Duane Champion to obtain a copy.
The laboratory still contains some of the original equipment used in these experiments, a tribute to curiosity, ingenuity, and science.
Paleomagnetism and Magnetic Techniques
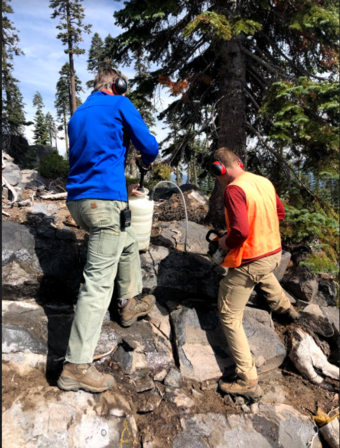
One of the more intriguing aspects of the geomagnetic field is its ability to reverse its polarity. It has been found that the north and south magnetic poles have switched places repeatedly throughout Earth's history and many of these reversals have been accurately dated. Research in the laboratory led to the development of the Geomagnetic Polarity Time Scale, which documents these reversals. The time scale provides a means of establishing the age of young geologic materials, including volcanic rocks, sedimentary deposits, and deep-sea cores. It has been used to date changes in the marine fossil record, the on-land mammalian fossil record, and was one of the means by which ages of early man sites in Africa were determined. The time scale also was used to calibrate marine magnetic anomaly time scales and is a valuable tool for establishing correlations between geologic formations.
In addition to the long-term geomagnetic field behavior that produces the polarity signal, significant short-term variations also occur. This latter behavior, occurring on timescales of hundreds of years, is known as Paleo-Secular Variation (PSV). PSV is responsible for the large magnetic anomalies that exist in certain areas of the world and contributes to the gradual movement of the magnetic poles. The north magnetic pole, for instance, has moved more than 500 miles since its position was first located in 1831. The PSV record contained in rapidly deposited sediments serves as an important correlation tool. Rapid changes in magnetic field direction (less than 0.5 degrees to more than 20 degrees per century) allows the differentiation of individual lava flows and archeological artifacts with a resolution that is unattainable with radiometric dating. The PSV signal provides a way to estimating recurrence interval and duration of volcanic eruptions and is an important consideration in the assessment of volcanic hazards.
Although the magnetic poles do drift on a short-term basis due to secular variation, averaging their positions over a period of several thousand years yields a mean direction corresponding to the location of the geographic poles (the Earth's spin axis). By determining the average magnetization of rock sequences on the stable parts of a continent for a given geologic period, an Apparent Polar Wander (APW) path can be constructed for that continent. Magnetic directions in rocks from less stable parts of a continental landmass, such as the Pacific Northwest, can be compared with the appropriate reference paleomagnetic poles from the APW to determine their rotation and/or translation relative to the continent as a whole. Pioneering research in the laboratory led to the realization that many rock assemblages ("suspect terranes") along the Pacific margin have been transported great distances from their place of origin. Because the Earth's spin axis changes little, if at all, an APW path also serves to document a continent's travels over geologic time. By comparing the APW paths from different continents, one can determine their past positions relative to one another.
The intensity of the geomagnetic field varies on time scales comparable to that of directional secular variation described above. Because the geomagnetic field provides shielding against incoming cosmic rays, its strength determines the amount of this radiation that reaches the upper atmosphere and the surface of the Earth. Reactions with these cosmic rays produces radioactive isotopes of certain elements such as 10Be, 14C, 36Cl, 3He, and others that are useful for dating and correlating geologic materials. Fluctuations in magnetic field strength, however, determine the amount of nuclides produced at any given time and uncertainties in nuclide production rates are a major factor affecting the accuracy of age determinations. By accurately determining geomagnetic paleointensity through time, the production rates of the cosmogenic nuclides can be established more closely, thus enhancing the various dating methods.
A measure of how well a material acquires an induced magnetization in response to a magnetic field is known as that material's magnetic susceptibility. This property can be very useful for geologic correlations. In a sedimentary sequence or core, for example, magnetic susceptibility will vary based on the amount of magnetic material present. A record of the variation in magnetic susceptibility with depth in a core can then serve as a "fingerprint" that can be compared with other nearby cores or sequences. This method can be very important in those materials that are too young to have recorded a polarity signature and where the secular variation signal may not be well expressed or is ambiguous. In certain areas, the magnetic susceptibility record has been found to be a proxy for the climatic record.
Anisotropy of Magnetic Susceptibility (AMS), how rock magnetic susceptibility varies along different axes, has applications for geologic studies. Although the susceptibility differences are quite small, they are easily measured. The minimum susceptibility direction has been shown to be perpendicular to the surface of lava flows and welded tuffs, and the maximum susceptibility direction to be parallel to the direction of lava or tuff flow. By determining the AMS at various sites within broadly distributed ash flow tuffs, researchers have been able to use the maximum susceptibility directions to determine the source areas of eruption. The same technique also can be used to determine current flow in sediments.