Coastal Resource Evaluation for Management Application (CREMA)
Coastal environments are dynamic systems that provide high ecological, economical, recreational, and cultural value. Managing coastal systems requires a comprehensive understanding of the complex interactions between geological and ecological processes, as well as the ability to predict both the near-term and long-term impacts of storms and sea-level rise. The Coastal Resource Evaluation for Management Application (CREMA) project is focused on understanding the integrated geomorphic, oceanographic and ecologic processes that transform coastal environments over varying temporal and spatial scales to inform natural (e.g., habitat, protected species, living coastal and marine resources) and cultural (e.g., historic buildings) resource management and associated needs. Our project team members and network of collaborators have expertise in oceanography, coastal geology, population ecology, landscape ecology, conservation biology, ecological modeling, decision analysis, and natural resource economics.
The objectives of the CREMA project are to:
- Characterize changes in the physical environment of coastal systems in response to long-term human alterations and climatic patterns (e.g. storms, sea-level change) through data collection and analysis
- Develop and apply predictive numerical models to assess coastal evolution due to natural (e.g., storms, sea-level rise) and human-induced (e.g., restoration) drivers
- Identify resulting habitat characteristics and evaluate how species usage may change in the future
- Apply structured decision making (also known as decision analysis) and adaptive management to assist natural resource managers with management decisions in coastal and marine systems
We have developed a framework that integrates data, analysis, and predictive models developed within the USGS Coastal and Marine Hazards and Resources Program and USGS Wetland and Aquatic Research Center (and available through other partnerships) to assess the cumulative effects of environmental drivers and management actions on coastal systems (Figure 1). By considering multiple objectives simultaneously and integrating multiple models of system behavior, we create an ecosystem-based-management approach that provides guidance and strategies for restoration efforts while also considering the uncertainty associated with future decisions.
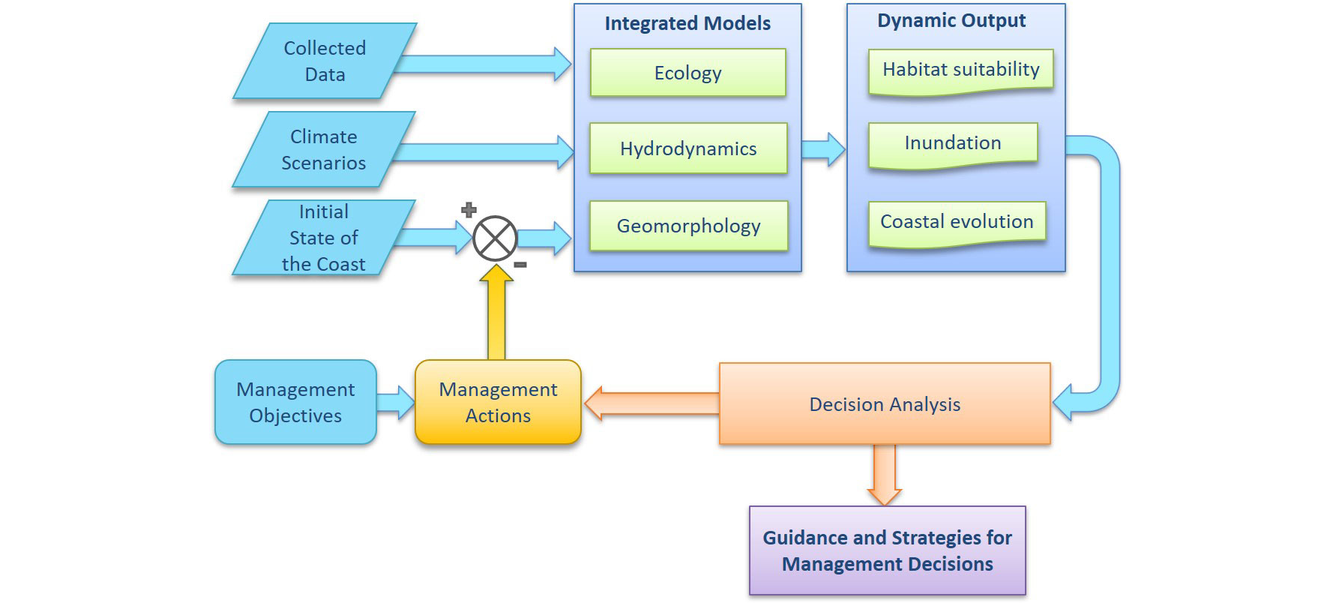
The CREMA project focuses on adaptation planning strategies to address physical and ecological coastal change processes in the following four areas:
Coastal Sediment Dynamics:
Barrier island systems perform a vital function in protecting mainland communities from storms, serve as critical habitat for numerous endangered species, support recreation, and stimulate economic growth through tourism. This physical environment is controlled by natural and human processes that affect sediment dynamics, punctuated by intense storm impacts that overwash the barrier islands and erode sand from the shoreface. These processes form a complex interrelationship between morphologic and oceanographic conditions that regulates the flux of sediment through the barrier island system. It is necessary to understand the sediment budget to establish base level conditions, identify erosional hotspots, and monitor coastal management efforts. This task characterizes the physical environment, identifies morphologic change, and models the sediment budget as a function of residual inventories over decadal timescales. This process captures island response to long-term human alteration and climatic patterns such as periods of increased storminess, which is more effective at assessing island resiliency than measuring change over a single storm impact. Understanding change to the physical environment over time is key to successfully predicting island response to future storm impacts, human alteration, and sea-level rise, which determine the fate of the ecology that the island supports. The impact of change on ecosystem function starts with establishing baselevel physical processes that are both generative and terminal. Predicting ecosystem tipping points is a necessary trigger for effective decision making and management response.
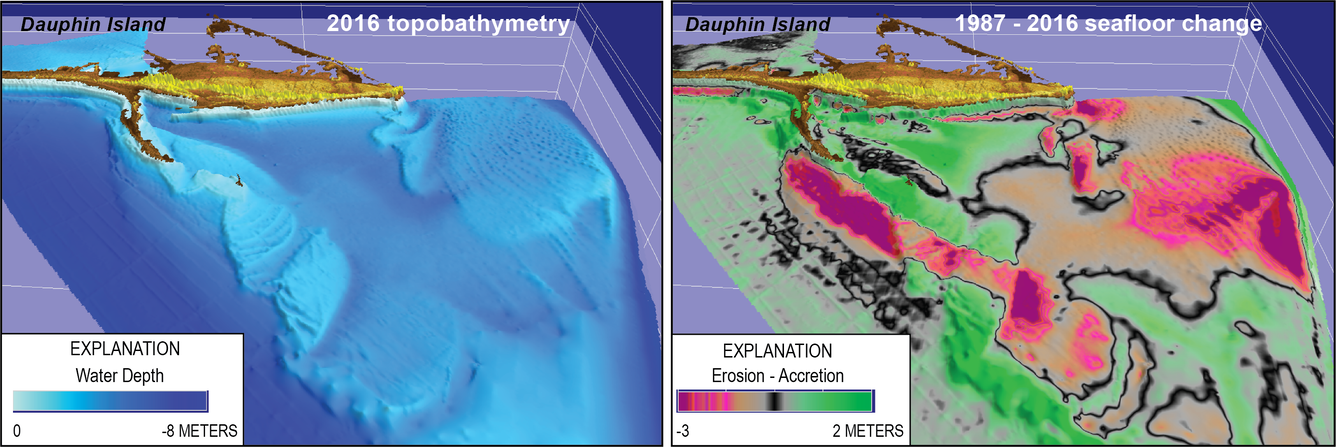
Coastal Evolution:
We develop and apply integrated hydrodynamic and morphodynamic models to simulate coastal processes including tides, waves, hurricane storm surge and coastal morphology. These numerical models allow us to understand how the coast has evolved historically and make predictions on how it may change in the future under short- and long-term drivers such as extreme storms and sea-level rise. This type of modeling can be used to inform management decision-making, such as assessing the effectiveness of potential restoration actions to enhance coastal resiliency.
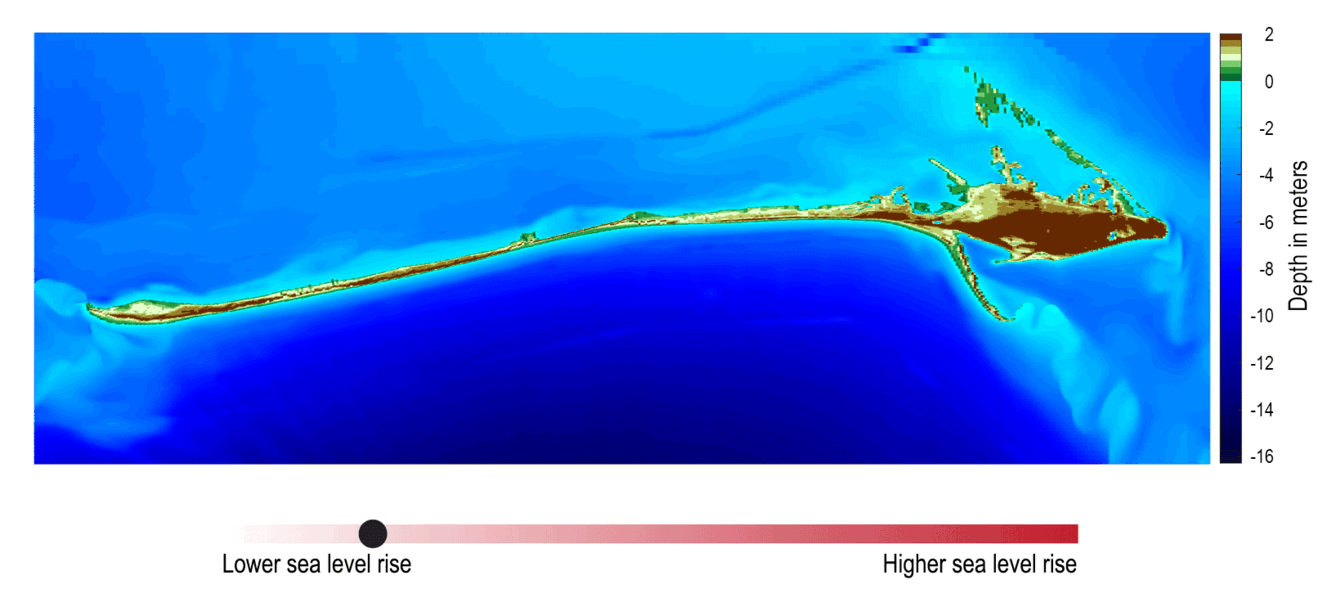
Species Use and Management:
We work with natural resource management partners to understand the needs of species of conservation concern. To support this work, we are developing a suite of habitat selection models (Bayesian networks) that estimate the probability that the species of interest will use a given geographical location, based on that location’s landcover characteristics. These probabilistic models explicitly convey prediction uncertainties in their results; can be used with remotely sensed imagery to map current or historical habitat availability (Figure 3); and can be linked to other predictive landscape models (e.g., see Coastal Evolution above) to indicate likely habitat availability under management, storm, and sea-level rise scenarios. To date, we’ve developed habitat models for nesting piping plovers as well as preliminary models for nesting sea turtles and wintering shorebirds.
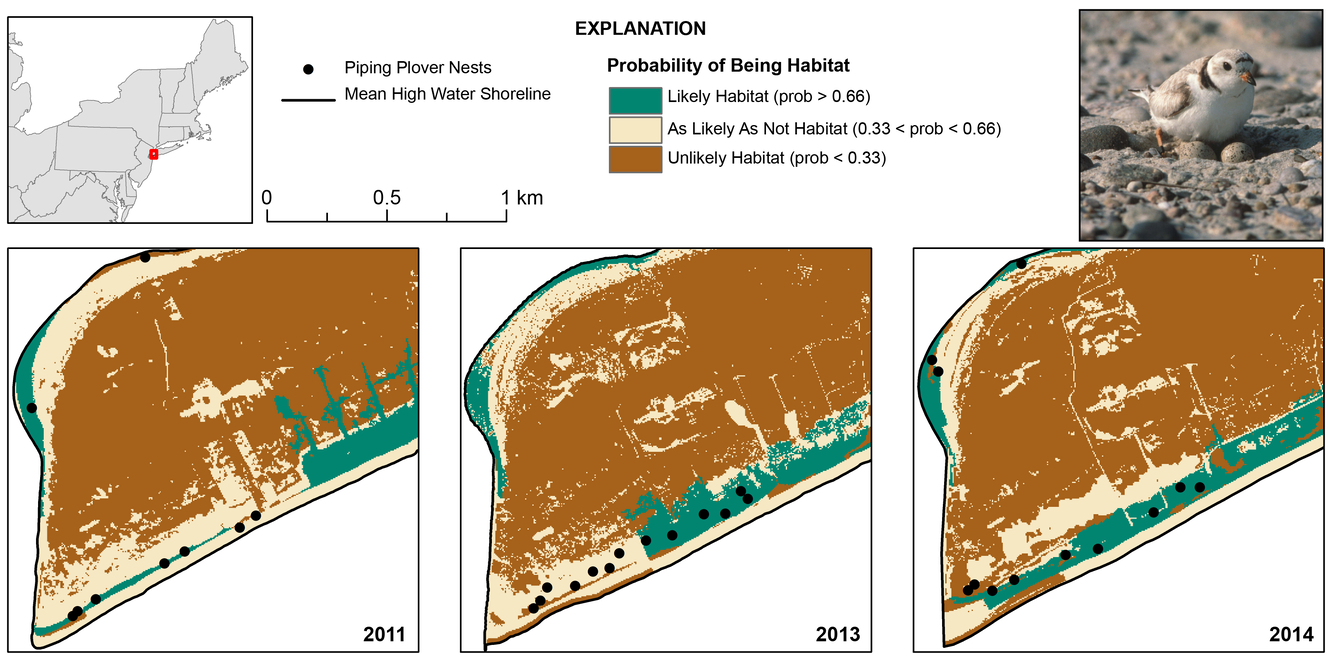
Structured decision making and adaptive management:
Our expertise include: (1) problem framing, stakeholder engagement, elicitation and quantification of objectives for natural resource management; (2) development of system models that project outcomes of restoration activities (e.g., ecological forecasting [population viability analysis]); (3) statistical modeling to parameterize system models (e.g., hierarchical models with Bayesian approaches (e.g., capture-mark-recapture, occupancy analyses)); (4) spatial optimization, such as optimal design of conservation reserve lands in response to global change processes (e.g., acquisition or divestment from parcels, Figure 5) and optimal allocation of restoration efforts in space and time (e.g., where and when to implement restoration activities such as beach nourishment, oyster reef and seagrass restoration); (5) formal application of adaptive management in natural resource decision-making; (6) consideration of the risk tolerance of decision makers.
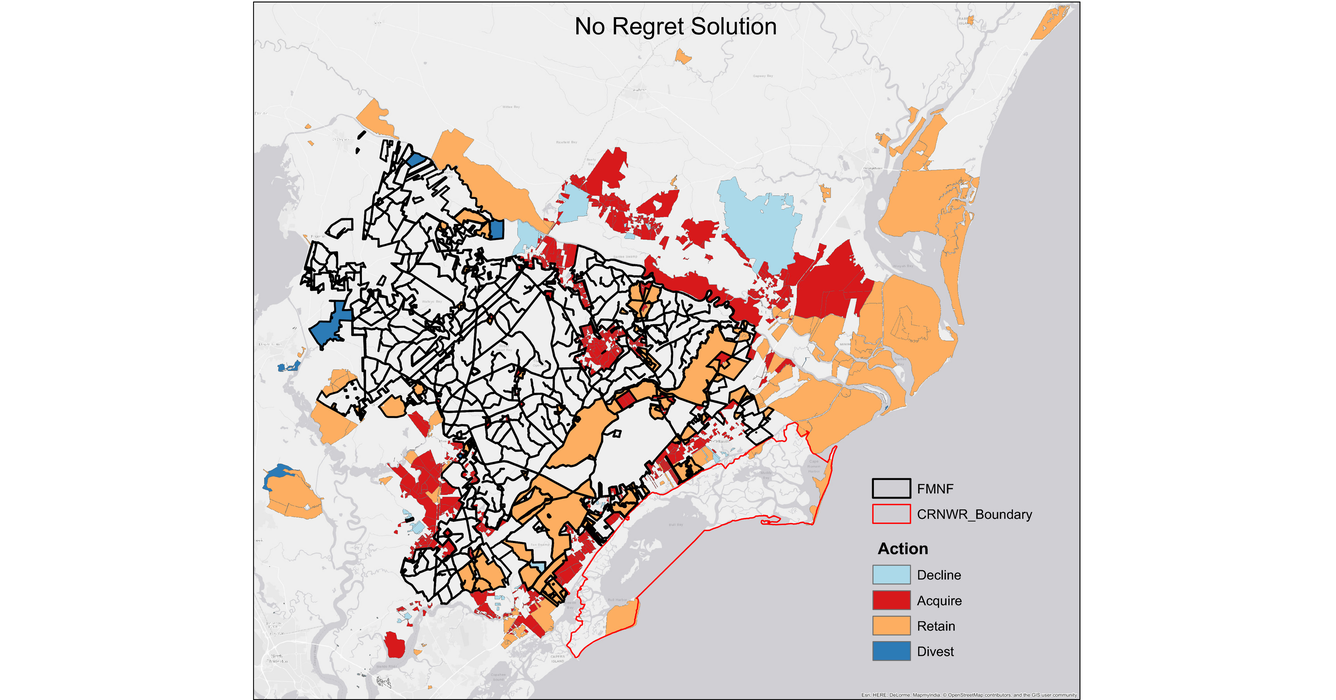
Our collaborative efforts include:
- NFWF Alabama Barrier Island Restoration Assessment (https://cesamusace.maps.arcgis.com/apps/MapSeries/index.html?appid=ea29cd4e1f3b432e8c520df3fb7a9f8b)
- NOAA ESLR Integrated Modeling of the Effects of Sea Level Rise Across Estuaries, Marshes and Barrier Islands in the Mississippi Sound (https://coastalscience.noaa.gov/project/integrated-modeling-of-the-effects-of-sea-level-rise-across-estuaries-marshes-and-barrier-islands-in-mississippi-sound/)
- NFWF Little Dauphin Island Restoration Assessment https://www.nfwf.org/sites/default/files/2020-04/al-little-dauphin-island-restoration-assessment-17.pdf
- RESTORE Enhancing Opportunities for Beneficial Use of Dredge Sediments - Grand Bay Mississippi Sound Back-Barrier Island Restoration Project Feasibility Study https://dwhprojecttracker.org/project-information/?project=1087
- NOAA ESLR Dynamic Sea Level Rise Assessments of the Ability of Natural and Nature-based Features to Mitigate Surge and Nuisance Flooding https://coastalscience.noaa.gov/project/sea-level-rise-assessments-mitigate-surge-flooding/
Below are other science projects associated with this project.
Alabama Barrier Island Restoration Assessment
Below are data or web applications associated with this project.
Coastal Multibeam Bathymetry Data Collected in 2019 off of Santa Rosa Island, Florida
Below are publications associated with this project.
Development and application of an empirical dune growth model for evaluating barrier island recovery from storms
Sensitivity of storm response to antecedent topography in the XBeach model
The roles of storminess and sea level rise in decadal barrier island evolution
Seafloor change around the Mississippi barrier islands, 1920 to 2016—The influence of storm effects on inlet and island morphodynamics
Spatial conservation planning under uncertainty: Adapting to climate change risks using modern portfolio theory
Below are news stories associated with this project.
Coastal environments are dynamic systems that provide high ecological, economical, recreational, and cultural value. Managing coastal systems requires a comprehensive understanding of the complex interactions between geological and ecological processes, as well as the ability to predict both the near-term and long-term impacts of storms and sea-level rise. The Coastal Resource Evaluation for Management Application (CREMA) project is focused on understanding the integrated geomorphic, oceanographic and ecologic processes that transform coastal environments over varying temporal and spatial scales to inform natural (e.g., habitat, protected species, living coastal and marine resources) and cultural (e.g., historic buildings) resource management and associated needs. Our project team members and network of collaborators have expertise in oceanography, coastal geology, population ecology, landscape ecology, conservation biology, ecological modeling, decision analysis, and natural resource economics.
The objectives of the CREMA project are to:
- Characterize changes in the physical environment of coastal systems in response to long-term human alterations and climatic patterns (e.g. storms, sea-level change) through data collection and analysis
- Develop and apply predictive numerical models to assess coastal evolution due to natural (e.g., storms, sea-level rise) and human-induced (e.g., restoration) drivers
- Identify resulting habitat characteristics and evaluate how species usage may change in the future
- Apply structured decision making (also known as decision analysis) and adaptive management to assist natural resource managers with management decisions in coastal and marine systems
We have developed a framework that integrates data, analysis, and predictive models developed within the USGS Coastal and Marine Hazards and Resources Program and USGS Wetland and Aquatic Research Center (and available through other partnerships) to assess the cumulative effects of environmental drivers and management actions on coastal systems (Figure 1). By considering multiple objectives simultaneously and integrating multiple models of system behavior, we create an ecosystem-based-management approach that provides guidance and strategies for restoration efforts while also considering the uncertainty associated with future decisions.
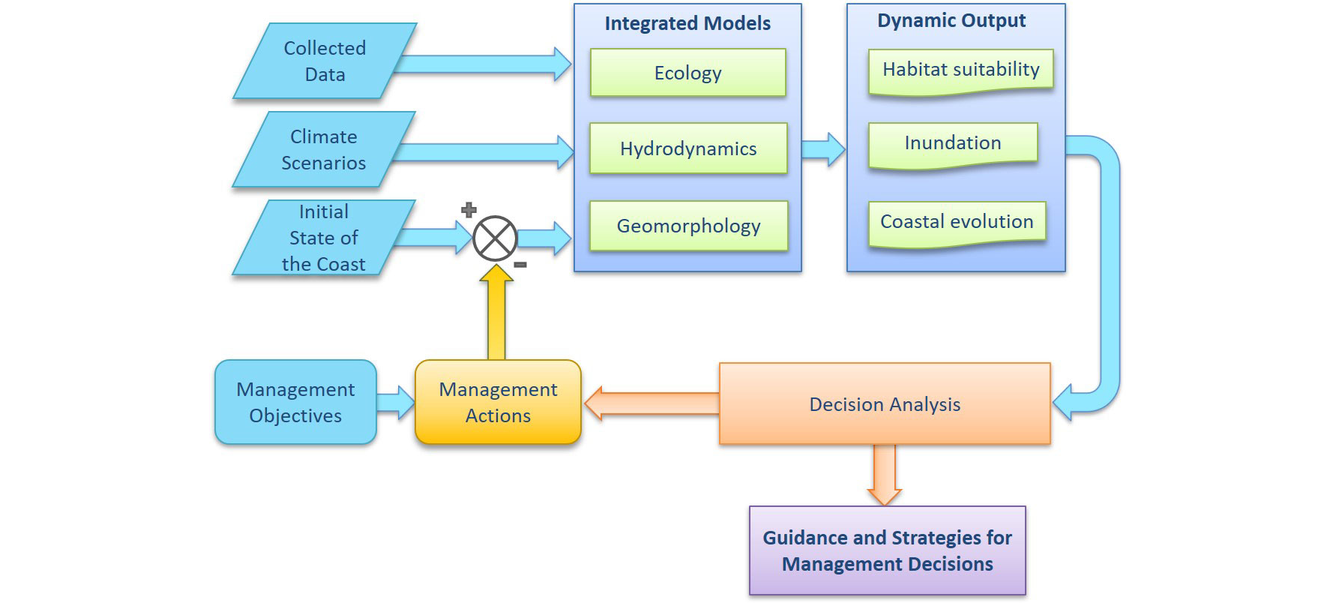
The CREMA project focuses on adaptation planning strategies to address physical and ecological coastal change processes in the following four areas:
Coastal Sediment Dynamics:
Barrier island systems perform a vital function in protecting mainland communities from storms, serve as critical habitat for numerous endangered species, support recreation, and stimulate economic growth through tourism. This physical environment is controlled by natural and human processes that affect sediment dynamics, punctuated by intense storm impacts that overwash the barrier islands and erode sand from the shoreface. These processes form a complex interrelationship between morphologic and oceanographic conditions that regulates the flux of sediment through the barrier island system. It is necessary to understand the sediment budget to establish base level conditions, identify erosional hotspots, and monitor coastal management efforts. This task characterizes the physical environment, identifies morphologic change, and models the sediment budget as a function of residual inventories over decadal timescales. This process captures island response to long-term human alteration and climatic patterns such as periods of increased storminess, which is more effective at assessing island resiliency than measuring change over a single storm impact. Understanding change to the physical environment over time is key to successfully predicting island response to future storm impacts, human alteration, and sea-level rise, which determine the fate of the ecology that the island supports. The impact of change on ecosystem function starts with establishing baselevel physical processes that are both generative and terminal. Predicting ecosystem tipping points is a necessary trigger for effective decision making and management response.
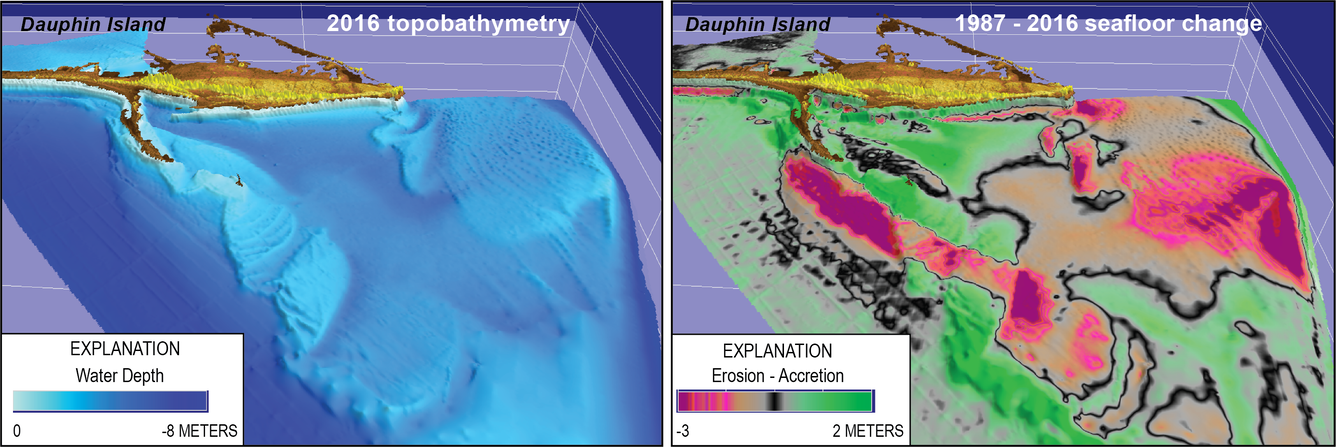
Coastal Evolution:
We develop and apply integrated hydrodynamic and morphodynamic models to simulate coastal processes including tides, waves, hurricane storm surge and coastal morphology. These numerical models allow us to understand how the coast has evolved historically and make predictions on how it may change in the future under short- and long-term drivers such as extreme storms and sea-level rise. This type of modeling can be used to inform management decision-making, such as assessing the effectiveness of potential restoration actions to enhance coastal resiliency.
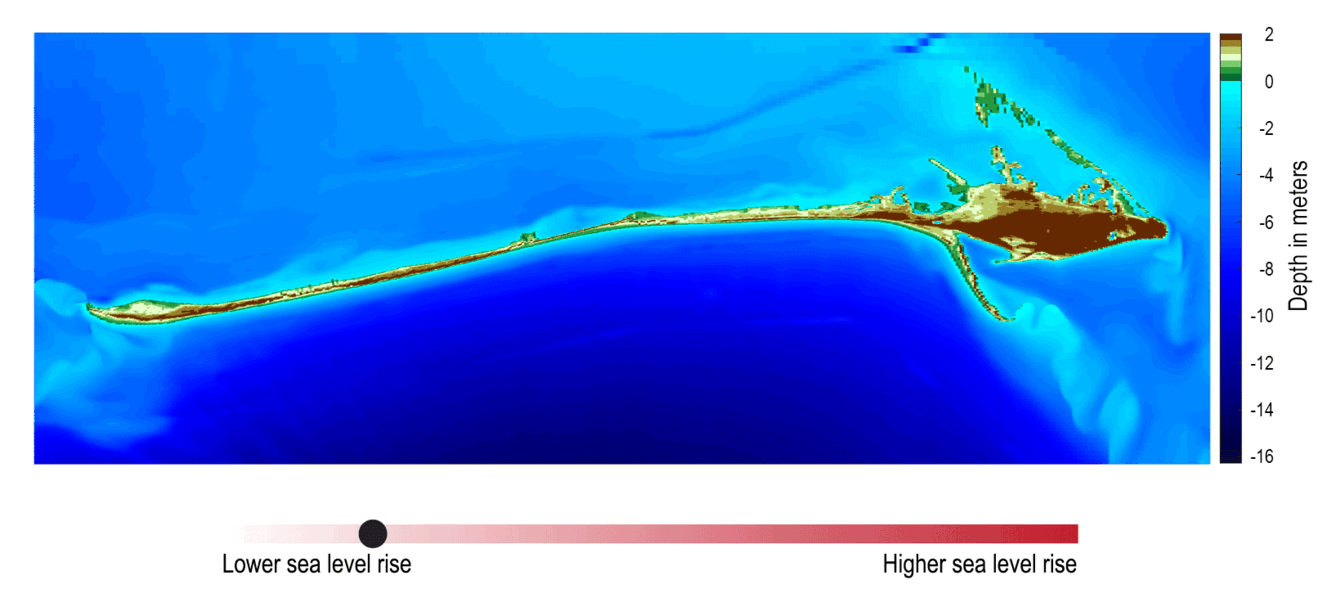
Species Use and Management:
We work with natural resource management partners to understand the needs of species of conservation concern. To support this work, we are developing a suite of habitat selection models (Bayesian networks) that estimate the probability that the species of interest will use a given geographical location, based on that location’s landcover characteristics. These probabilistic models explicitly convey prediction uncertainties in their results; can be used with remotely sensed imagery to map current or historical habitat availability (Figure 3); and can be linked to other predictive landscape models (e.g., see Coastal Evolution above) to indicate likely habitat availability under management, storm, and sea-level rise scenarios. To date, we’ve developed habitat models for nesting piping plovers as well as preliminary models for nesting sea turtles and wintering shorebirds.
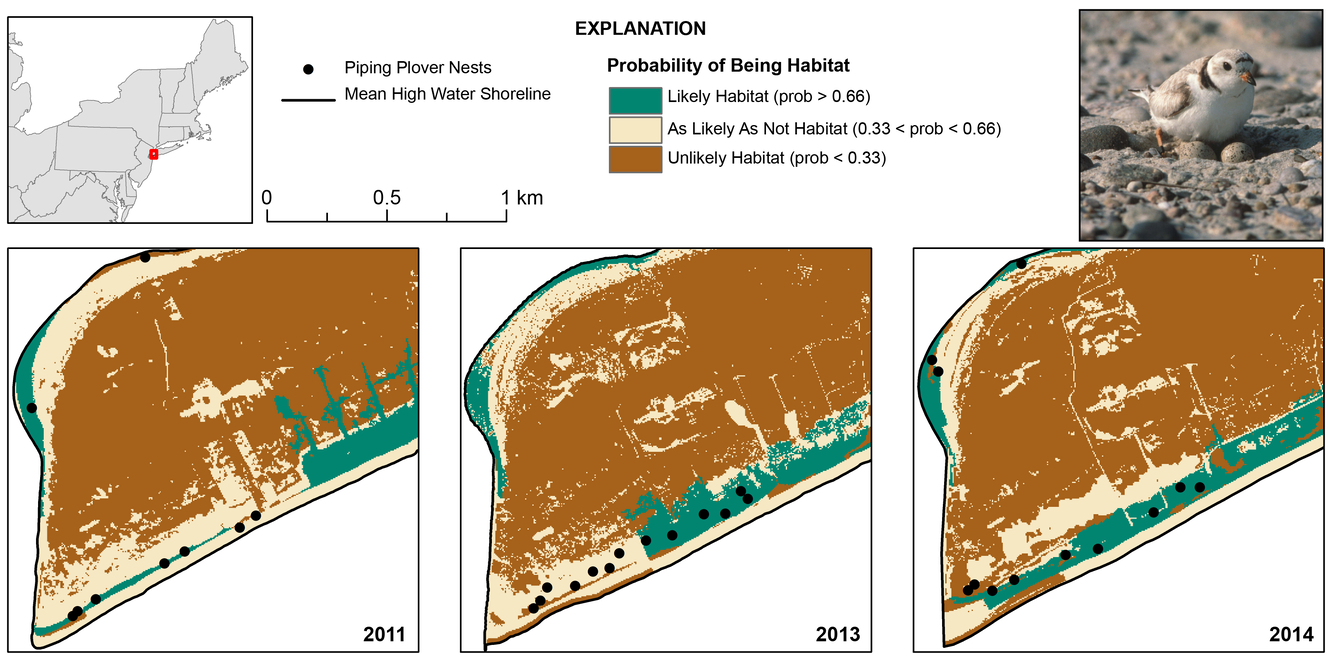
Structured decision making and adaptive management:
Our expertise include: (1) problem framing, stakeholder engagement, elicitation and quantification of objectives for natural resource management; (2) development of system models that project outcomes of restoration activities (e.g., ecological forecasting [population viability analysis]); (3) statistical modeling to parameterize system models (e.g., hierarchical models with Bayesian approaches (e.g., capture-mark-recapture, occupancy analyses)); (4) spatial optimization, such as optimal design of conservation reserve lands in response to global change processes (e.g., acquisition or divestment from parcels, Figure 5) and optimal allocation of restoration efforts in space and time (e.g., where and when to implement restoration activities such as beach nourishment, oyster reef and seagrass restoration); (5) formal application of adaptive management in natural resource decision-making; (6) consideration of the risk tolerance of decision makers.
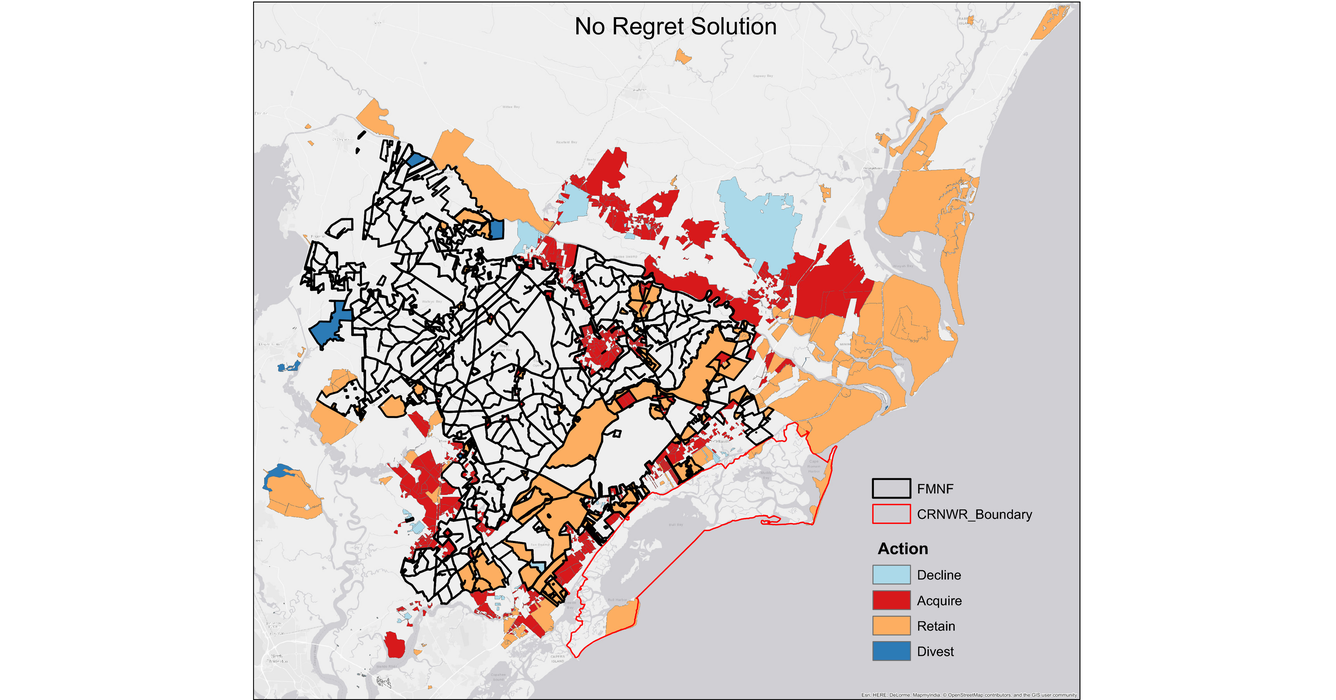
Our collaborative efforts include:
- NFWF Alabama Barrier Island Restoration Assessment (https://cesamusace.maps.arcgis.com/apps/MapSeries/index.html?appid=ea29cd4e1f3b432e8c520df3fb7a9f8b)
- NOAA ESLR Integrated Modeling of the Effects of Sea Level Rise Across Estuaries, Marshes and Barrier Islands in the Mississippi Sound (https://coastalscience.noaa.gov/project/integrated-modeling-of-the-effects-of-sea-level-rise-across-estuaries-marshes-and-barrier-islands-in-mississippi-sound/)
- NFWF Little Dauphin Island Restoration Assessment https://www.nfwf.org/sites/default/files/2020-04/al-little-dauphin-island-restoration-assessment-17.pdf
- RESTORE Enhancing Opportunities for Beneficial Use of Dredge Sediments - Grand Bay Mississippi Sound Back-Barrier Island Restoration Project Feasibility Study https://dwhprojecttracker.org/project-information/?project=1087
- NOAA ESLR Dynamic Sea Level Rise Assessments of the Ability of Natural and Nature-based Features to Mitigate Surge and Nuisance Flooding https://coastalscience.noaa.gov/project/sea-level-rise-assessments-mitigate-surge-flooding/
Below are other science projects associated with this project.
Alabama Barrier Island Restoration Assessment
Below are data or web applications associated with this project.
Coastal Multibeam Bathymetry Data Collected in 2019 off of Santa Rosa Island, Florida
Below are publications associated with this project.
Development and application of an empirical dune growth model for evaluating barrier island recovery from storms
Sensitivity of storm response to antecedent topography in the XBeach model
The roles of storminess and sea level rise in decadal barrier island evolution
Seafloor change around the Mississippi barrier islands, 1920 to 2016—The influence of storm effects on inlet and island morphodynamics
Spatial conservation planning under uncertainty: Adapting to climate change risks using modern portfolio theory
Below are news stories associated with this project.